Why Are Autism Rates Steadily Rising?
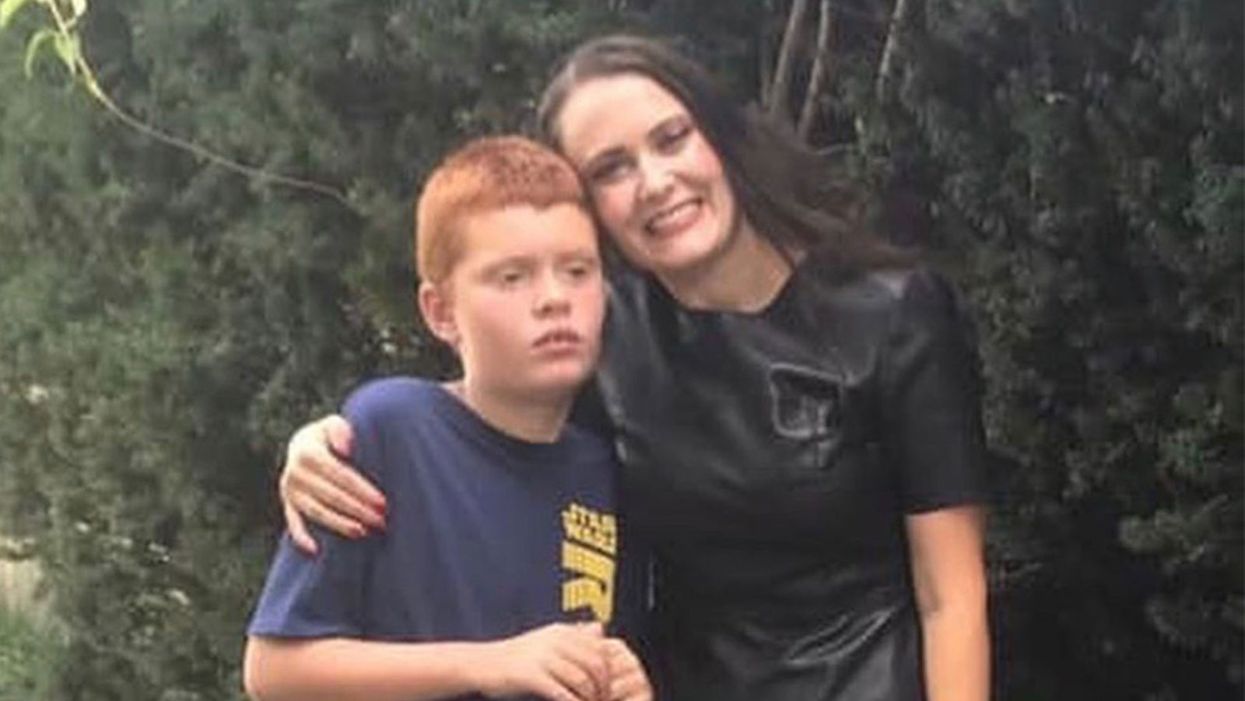
Stefania Sterling with her son Charlie, who was diagnosed at age 3 with autism.
Stefania Sterling was just 21 when she had her son, Charlie. She was young and healthy, with no genetic issues apparent in either her or her husband's family, so she expected Charlie to be typical.
"It is surprising that the prevalence of a significant disorder like autism has risen so consistently over a relatively brief period."
It wasn't until she went to a Mommy and Me music class when he was one, and she saw all the other one-year-olds walking, that she realized how different her son was. He could barely crawl, didn't speak, and made no eye contact. By the time he was three, he was diagnosed as being on the lower functioning end of the autism spectrum.
She isn't sure why it happened – and researchers, too, are still trying to understand the basis of the complex condition. Studies suggest that genes can act together with influences from the environment to affect development in ways that lead to Autism Spectrum Disorder (ASD). But rates of ASD are rising dramatically, making the need to figure out why it's happening all the more urgent.
The Latest News
Indeed, the CDC's latest autism report, released last week, which uses 2016 data, found that the prevalence of ASD in four-year-old children was one in 64 children, or 15.6 affected children per 1,000. That's more than the 14.1 rate they found in 2014, for the 11 states included in the study. New Jersey, as in years past, was the highest, with 25.3 per 1,000, compared to Missouri, which had just 8.8 per 1,000.
The rate for eight-year-olds had risen as well. Researchers found the ASD prevalence nationwide was 18.5 per 1,000, or one in 54, about 10 percent higher than the 16.8 rate found in 2014. New Jersey, again, was the highest, at one in 32 kids, compared to Colorado, which had the lowest rate, at one in 76 kids. For New Jersey, that's a 175 percent rise from the baseline number taken in 2000, when the state had just one in 101 kids.
"It is surprising that the prevalence of a significant disorder like autism has risen so consistently over a relatively brief period," said Walter Zahorodny, an associate professor of pediatrics at Rutgers New Jersey Medical School, who was involved in collecting the data.
The study echoed the findings of a surprising 2011 study in South Korea that found 1 in every 38 students had ASD. That was the the first comprehensive study of autism prevalence using a total population sample: A team of investigators from the U.S., South Korea, and Canada looked at 55,000 children ages 7 to 12 living in a community in South Korea and found that 2.64 percent of them had some level of autism.
Searching for Answers
Scientists can't put their finger on why rates are rising. Some say it's better diagnosis. That is, it's not that more people have autism. It's that we're better at detecting it. Others attribute it to changes in the diagnostic criteria. Specifically, the May 2013 update of the Diagnostic and Statistical Manual of Mental Disorders-5 -- the standard classification of mental disorders -- removed the communication deficit from the autism definition, which made more children fall under that category. Cynical observers believe physicians and therapists are handing out the diagnosis more freely to allow access to services available only to children with autism, but that are also effective for other children.
Alycia Halladay, chief science officer for the Autism Science Foundation in New York, said she wishes there were just one answer, but there's not. While she believes the rising ASD numbers are due in part to factors like better diagnosis and a change in the definition, she does not believe that accounts for the entire rise in prevalence. As for the high numbers in New Jersey, she said the state has always had a higher prevalence of autism compared to other states. It is also one of the few states that does a good job at recording cases of autism in its educational records, meaning that children in New Jersey are more likely to be counted compared to kids in other states.
"Not every state is as good as New Jersey," she said. "That accounts for some of the difference compared to elsewhere, but we don't know if it's all of the difference in prevalence, or most of it, or what."
"What we do know is that vaccinations do not cause autism."
There is simply no defined proven reason for these increases, said Scott Badesch, outgoing president and CEO of the Autism Society of America.
"There are suggestions that it is based on better diagnosis, but there are also suggestions that the incidence of autism is in fact increasing due to reasons that have yet been determined," he said, adding, "What we do know is that vaccinations do not cause autism."
Zahorodny, the pediatrics professor, believes something is going on beyond better detection or evolving definitions.
"Changes in awareness and shifts in how children are identified or diagnosed are relevant, but they only take you so far in accounting for an increase of this magnitude," he said. "We don't know what is driving the surge in autism recorded by the ADDM Network and others."
He suggested that the increase in prevalence could be due to non-genetic environmental triggers or risk factors we do not yet know about, citing possibilities including parental age, prematurity, low birth rate, multiplicity, breech presentation, or C-section delivery. It may not be one, but rather several factors combined, he said.
"Increases in ASD prevalence have affected the whole population, so the triggers or risks must be very widely dispersed across all strata," he added.
There are studies that find new risk factors for ASD almost on a daily basis, said Idan Menashe, assistant professor in the Department of Health at Ben-Gurion University of the Negev, the fastest growing research university in Israel.
"There are plenty of studies that find new genetic variants (and new genes)," he said. In addition, various prenatal and perinatal risk factors are associated with a risk of ASD. He cited a study his university conducted last year on the relationship between C-section births and ASD, which found that exposure to general anesthesia may explain the association.
Whatever the cause, health practitioners are seeing the consequences in real time.
"People say rates are higher because of the changes in the diagnostic criteria," said Dr. Roseann Capanna-Hodge, a psychologist in Ridgefield, CT. "And they say it's easier for children to get identified. I say that's not the truth and that I've been doing this for 30 years, and that even 10 years ago, I did not see the level of autism that I do see today."
Sure, we're better at detecting autism, she added, but the detection improvements have largely occurred at the low- to mid- level part of the spectrum. The higher rates of autism are occurring at the more severe end, in her experience.
A Polarizing Theory
Among the more controversial risk factors scientists are exploring is the role environmental toxins may play in the development of autism. Some scientists, doctors and mental health experts suspect that toxins like heavy metals, pesticides, chemicals, or pollution may interrupt the way genes are expressed or the way endocrine systems function, manifesting in symptoms of autism. But others firmly resist such claims, at least until more evidence comes forth. To date, studies have been mixed and many have been more associative than causative.
"Today, scientists are still trying to figure out whether there are other environmental changes that can explain this rise, but studies of this question didn't provide any conclusive answer," said Menashe, who also serves as the scientific director of the National Autism Research Center at BGU.
"It's not everything that makes Charlie. He's just like any other kid."
That inconclusiveness has not dissuaded some doctors from taking the perspective that toxins do play a role. "Autism rates are rising because there is a mismatch between our genes and our environment," said Julia Getzelman, a pediatrician in San Francisco. "The majority of our evolution didn't include the kinds of toxic hits we are experiencing. The planet has changed drastically in just the last 75 years –- it has become more and more polluted with tens of thousands of unregulated chemicals being used by industry that are having effects on our most vulnerable."
She cites BPA, an industrial chemical that has been used since the 1960s to make certain plastics and resins. A large body of research, she says, has shown its impact on human health and the endocrine system. BPA binds to our own hormone receptors, so it may negatively impact the thyroid and brain. A study in 2015 was the first to identify a link between BPA and some children with autism, but the relationship was associative, not causative. Meanwhile, the Food and Drug Administration maintains that BPA is safe at the current levels occurring in food, based on its ongoing review of the available scientific evidence.
Michael Mooney, President of St. Louis-based Delta Genesis, a non-profit organization that treats children struggling with neurodevelopmental delays like autism, suspects a strong role for epigenetics, which refers to changes in how genes are expressed as a result of environmental influences, lifestyle behaviors, age, or disease states.
He believes some children are genetically predisposed to the disorder, and some unknown influence or combination of influences pushes them over the edge, triggering epigenetic changes that result in symptoms of autism.
For Stefania Sterling, it doesn't really matter how or why she had an autistic child. That's only one part of Charlie.
"It's not everything that makes Charlie," she said. "He's just like any other kid. He comes with happy moments. He comes with sad moments. Just like my other three kids."
Where Are the Lab-Grown Replacement Organs?
A futuristic rendering of a scientist holding a pair of lungs in a glass dish.
The headline blared from newspapers all the way back in 2006: "First Lab-Grown Organs Implanted in Humans!" A team from Wake Forest University had biopsied cells from the bladders of patients with spina bifida and used them to create brand new full-size bladders, which they then implanted. Although the bladders had to be emptied via catheter, they were still functioning a few years after implantation, and the public grew confident that doctors had climbed an intermediary step on the way to the medicine of science fiction. Ten years later, though, more than 20 people a day are still dying while waiting for an organ transplant, which leads to a simple question: Where are our fake organs?
"We can make small organs and tissues but we can't make larger ones."
Not coming anytime soon, unfortunately. The company that was created to transition Wake Forest's bladders to the market failed. And while there are a few simple bioengineered skins and cartilages already on the market, they are hardly identical to the real thing. Something like a liver could take another 20 to 25 years, says Shay Soker, professor at Wake Forest's Institute for Regenerative Medicine. "The first barrier is the technology: We can make small organs and tissues but we can't make larger ones," he says. "Also there are several cell types or functions that you can reliably make from stem cells, but not all of them, so the technology of stem cells has to catch up with what the body can do." Finally, he says, you have support the new organ inside the body, providing it with a circulatory and nervous system and integrating it with the immune system.
While these are all challenging problems, circulation appears to be the most intractable. "Tissue's not able to survive if the cells don't have oxygen, and the bigger it gets, the more complex vasculature you need to keep that alive," says Chiara Ghezzi, research professor in the Tufts University Department of Biomedical Engineering. "Vasculature is highly organized in the body. It has a hierarchical structure, with different branches that have different roles depending on where they are." So far, she says, researchers have had trouble scaling up from capillaries to larger vessels that could be grafted onto blood vessels in a patient's body.
"The FDA is still getting its hands and minds around the field of tissue engineering."
Last, but hardly least, is the question of FDA approval. Lab-grown organs are neither drugs nor medical devices, and the agency is not set up to quickly or easily approve new technologies that don't fit into current categories. "The FDA is still getting its hands and minds around the field of tissue engineering," says Soker. "They were not used to that… so it requires the regulatory and financial federal agencies to really help and support these initiatives."
A pencil eraser-size model of the human brain is now being used for drug development and research.
If all of this sounds discouraging, it's worth mentioning some of the incredible progress the field has made since the first strides toward lab-grown organs began nearly 30 years ago: Though full-size replacement organs are still decades away, many labs have diverted their resources into what they consider an intermediate step, developing miniature organs and systems that can be used for drug development and research. This platform will yield more relevant results (Imagine! Testing cardiovascular drugs on an actual human heart!) and require the deaths of far fewer animals. And it's already here: Two years ago, scientists at Ohio State University developed a pencil eraser-size model of the human brain they intend to use for this exact purpose.
Perhaps the most exciting line of research these days is one that at first doesn't seem to have anything to do with bioengineered organs at all. Along with his colleagues, Chandan Sen, Director of the Center for Regenerative Medicine and Cell-based Therapies at Ohio State University, has developed a nanoscale chip that can turn any cell in the body into any other kind of cell—reverting fully differentiated adult cells into, essentially, stem cells, which can then grow into any tissue you want. Sen has used his chip to reprogram skin cells in the bodies of mice into neurons to help them recover from strokes, and blood vessels to save severe leg injuries. "There's this concept of a bioreactor, where you convince an organ to grow outside the body. They're getting more and more sophisticated over time. But to my mind it will never match the sophistication or complexity of the human body," Sen says. "I believe that in order to have an organ that behaves the way you want it to in the live body, you must use the body itself as a bioreactor, not a bunch of electronic gadgetry." There you have it, the next step in artificial organ manufacture is as crazy as it is intuitive: Grow it back where it was in the first place.
The Good, the Bad, and the Ugly in Personalized Medicine
Precision Medicine concept
Is the value of "personalized medicine" over-promised? Why is the quality of health care declining for many people despite the pace of innovation? Do patients and doctors have conflicting priorities? What is the best path forward?
"How do we generate evidence for value, which is what everyone is asking for?"
Some of the country's leading medical experts recently debated these questions at the prestigious annual Personalized Medicine Conference, held at Harvard Medical School in Boston, and LeapsMag was there to bring you the inside scoop.
Personalized Medicine: Is It Living Up to the Hype?
The buzzworthy phrase "personalized medicine" has been touted for years as the way of the future—customizing care to patients based on their predicted responses to treatments given their individual genetic profiles or other analyses. Since the initial sequencing of the human genome around fifteen years ago, the field of genomics has exploded as the costs have dramatically come down – from $2.7 billion to $1000 or less today. Given cheap access to such crucial information, the medical field has been eager to embrace an ultramodern world in which preventing illnesses is status quo, and treatments can be tailored for maximum effectiveness. But whether that world has finally arrived remains debatable.
"I've been portrayed as an advocate for genomics, because I'm excited about it," said Robert C. Green, Director of the Genomes2People Research Program at Harvard Medical School, the Broad Institute, and Brigham and Women's Hospital. He qualified his advocacy by saying that he tries to remain 'equipoised' or balanced in his opinions about the future of personalized medicine, and expressed skepticism about some aspects of its rapid commercialization.
"I have strong feelings about some of the [precision medicine] products that are rushing out to market in both the physician-mediated space and the consumer space," Green said, and challenged the value and sustainability of these products, such as their clinical utility and ability to help produce favorable health outcomes. He asked what most patients and providers want to know, which is, "What are the medical, behavioral, and economic outcomes? How do we generate evidence for value, which is what everyone is asking for?" He later questioned whether the use of 'sexy' and expensive diagnostic technologies is necessarily better than doing things the old-fashioned way. For instance, it is much easier and cheaper to ask a patient directly about their family history of disease, instead of spending thousands of dollars to obtain the same information with pricey diagnostic tests.
"Our mantra is to try to do data-driven health...to catch disease when it occurs early."
Michael Snyder, Professor & Chair of the Department of Genetics and Director of the Center for Genomics and Personalized Medicine at Stanford University, called himself more of an 'enthusiast' about precision medicine products like wearable devices that can digitally track vital signs, including heart rate and blood oxygen levels. "I'm certainly not equipoised," he said, adding, "Our mantra is to try to do data-driven health. We are using this to try to understand health and catch disease when it occurs early."
Snyder then shared his personal account about how his own wearable device alerted him to seek treatment while he was traveling in Norway. "My blood oxygen was low and my heart rate was high, so that told me something was up," he shared. After seeing a doctor, he discovered he was suffering from Lyme disease. He then shared other similar success stories about some of the patients in his department. Using wearable health sensors, he said, could significantly reduce health care costs: "$245 billion is spent every year on diabetes, and if we reduce that by ten percent we just saved $24 billion."
From left, Robert Green, Michael Snyder, Sandro Galea, and Thomas Miller.
(Courtesy Rachele Hendricks-Sturrup)
A Core Reality: Unresolved Societal Issues
Sandro Galea, Dean and Professor at Boston University's School of Public Health, coined himself as a 'skeptic' but also an 'enormous fan' of new technologies. He said, "I want to make sure that you all [the audience] have the best possible treatment for me when I get sick," but added, "In our rush and enthusiasm to embrace personalized and precision medicine approaches, we have done that at the peril of forgetting a lot of core realities."
"There's no one to pay for health care but all of us."
Galea stressed the need to first address certain difficult societal issues because failing to do so will deter precision medicine cures in the future. "Unless we pay attention to domestic violence, housing, racism, poor access to care, and poverty… we are all going to lose," he said. Then he quoted recent statistics about the country's growing gap in both health and wealth, which could potentially erode patient and provider interest in personalized medicine.
Thomas Miller, the founder and partner of a venture capital firm dedicated to advancing precision medicine, agreed with Galea and said that "there's no one to pay for health care but all of us." He recalled witnessing 'abuse' of diagnostic technologies that he had previously invested in. "They were often used as mechanisms to provide unnecessary care rather than appropriate care," he said. "The trend over my 30-year professional career has been that of sensitivity over specificity."
In other words: doctors rely too heavily on diagnostic tools that are sensitive enough to detect signs of a disease, but not accurate enough to confirm the presence of a specific disease. "You will always find that you're sick from something," Miller said. He lamented the counter-productivity and waste brought on by such 'abuse' and added, "That's money that could be used to address some of the problems that you [Galea] just talked about."
Do Patients and Providers Have Conflicting Priorities?
Distrust in the modern health care system is not new in the United States. That fact that medical errors were the third leading cause of death in 2016 may have fueled this mistrust even more. And the level of mistrust appears correlated with race; a recent survey of 118 adults between 18 to 75 years old showed that black respondents were less likely to trust their doctors than the non-Hispanic white respondents. The black respondents were also more concerned about personal privacy and potentially harmful hospital experimentation.
"The vast majority of physicians in this country are incentivized to keep you sick."
As if this context weren't troubling enough, some of the panelists suggested that health care providers and patients have misaligned goals, which may be financially driven.
For instance, Galea stated that health care is currently 'curative' even though that money is better spent on prevention versus cures. "The vast majority of physicians in this country are incentivized to keep you sick," he declared. "They are paid by sick patient visits. Hospital CEOs are paid by the number of sick people they have in their beds." He highlighted this issue as a national priority and mentioned some case studies showing that the behaviors of hospital CEOs quickly change when payment is based on the number of patients in beds versus the number of patients being kept out of the beds. Green lauded Galea's comment as "good sense."
Green also cautioned the audience about potential financial conflicts of interest held by proponents of precision medicine technologies. "Many of the people who are promoting genomics and personalized medicine are people who have financial interests in that arena," he warned. He emphasized that those who are perhaps curbing the over-enthusiasm do not have financial interests at stake.
What is the Best Path Forward for Personalized Medicine?
As useful as personalized medicine may be for selecting the best course of treatment, there is also the flip side: It can allow doctors to predict who will not respond well—and this painful reality must be acknowledged.
Miller argued, "We have a duty to call out therapies that won't work, that will not heal, that need to be avoided, and that will ultimately lead to you saying to a patient, 'There is nothing for you that will work.'"
Although that may sound harsh, it captures the essence of this emerging paradigm, which is to maximize health by using tailored methods that are based on comparative effectiveness, evidence of outcomes, and patient preferences. After all, as Miller pointed out, it wouldn't do much good to prescribe someone a regimen with little reason to think it might help.
For the hype around personalized medicine to be fully realized, Green concluded, "We have to prove to people that [the value of it] is true."