7 New Insights about the Frontrunner U.S. Vaccine Candidate
Kira Peikoff was the editor-in-chief of Leaps.org from 2017 to 2021. As a journalist, her work has appeared in The New York Times, Newsweek, Nautilus, Popular Mechanics, The New York Academy of Sciences, and other outlets. She is also the author of four suspense novels that explore controversial issues arising from scientific innovation: Living Proof, No Time to Die, Die Again Tomorrow, and Mother Knows Best. Peikoff holds a B.A. in Journalism from New York University and an M.S. in Bioethics from Columbia University. She lives in New Jersey with her husband and two young sons. Follow her on Twitter @KiraPeikoff.
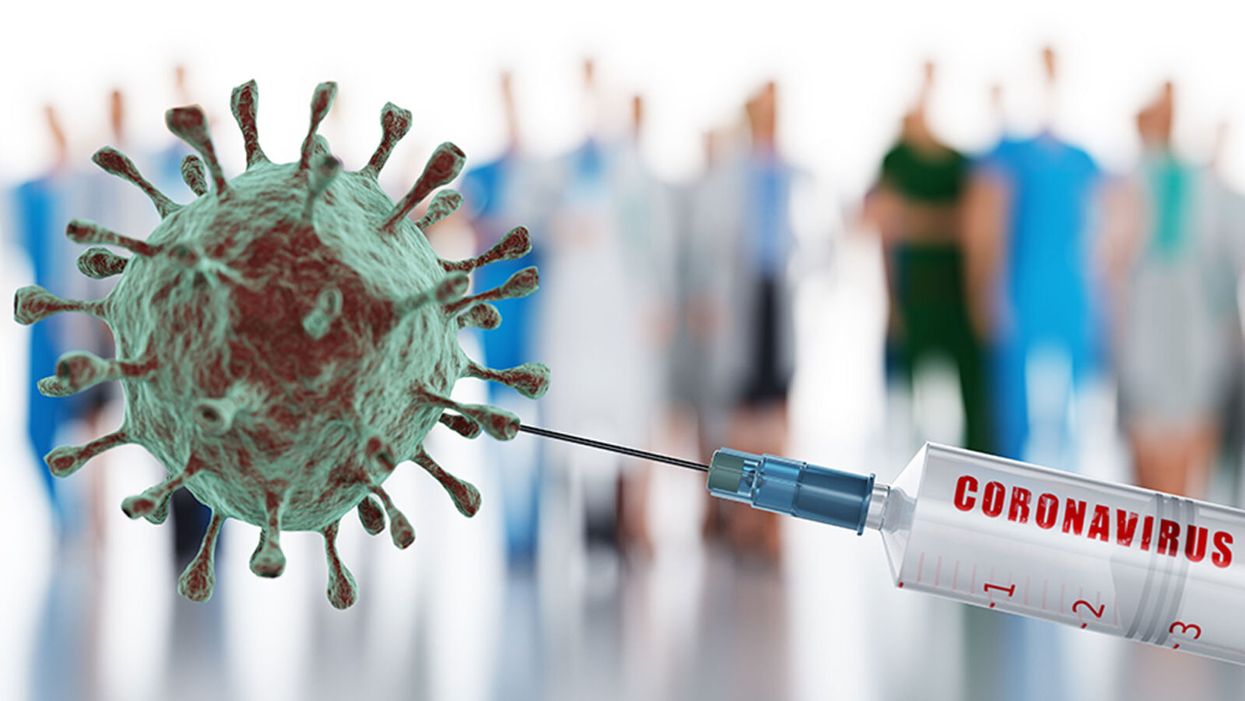
A vaccine from Moderna could be available as early as January, Fauci recently said.
Earlier this year, biotech company Moderna broke world records for speed in vaccine development. Their researchers translated the genetic code of the coronavirus into a vaccine candidate in just 42 days.
We're about to expand our safety data in Phase II.
Phase I of the clinical trial started in Seattle on March 16th, with the already-iconic image of volunteer Jennifer Haller calmly receiving the very first dose.
Instead of traditional methods, this vaccine uses a new -- and so far unproven -- technology based on synthetic biology: It hijacks the software of life – messenger RNA – to deliver a copy of the virus's genetic sequence into cells, which, in theory, triggers the body to produce antibodies to fight off a coronavirus infection.
U.S. National Institute of Allergy and Infectious Diseases Director Anthony Fauci called the vaccine's preclinical data "impressive" and told National Geographic this week that a vaccine could be ready for general use as early as January.
The Phase I trial has dosed 45 healthy adults. Phase II trials are about to start, enrolling around 600 adults. Pivotal efficacy trials would follow soon thereafter, bankrolled in collaboration with the government office BARDA (Biomedical Advanced Research and Development Authority).
Today, the chief medical officer of Moderna, Tal Zaks, answered burning questions from the public in a webinar hosted by STAT. Here's an edited and condensed summary of his answers.
1) When will a vaccine become available?
We expect to have data in early summer about the antibody levels from our mRNA vaccine. At the same time, we can measure the antibody levels of people who have had the disease, and we should be able to measure the ability of those antibodies to prevent disease.
We will not yet know if the mRNA vaccine works to prevent disease, but we could soon talk about a potential for benefit. We don't yet know about risk. We're about to expand our safety data in Phase II.
In the summer, there is an expectation that we will be launching pivotal trials, in collaboration with government agencies that are helping fund the research. The trials would be launched with the vaccine vs. a placebo with the goal of establishing: How many cases can we show we prevented with the vaccine?
This is determined by two factors: How big is the trial? And what's the attack rate in the population we vaccinate? The challenge will be to vaccinate in the areas where the risk of infection is still high in the coming months, and we're able to vaccinate and demonstrate fewer infections compared to a placebo. If the disease is happening faster in a given area, you will be able to see an outcome faster. Potentially by the end of the year, we will have the data to say if the vaccine works.
Will that be enough for regulatory approval? The main question is: When will we cross the threshold for the anticipated benefit of a presumed vaccine to be worth the risk?
There is a distinction between approval for those who need it most, like the elderly. Their unmet need and risk/benefit is not the same as it is for younger adults.
My private opinion: I don't think it's a one-size-fits-all. It will be a more measured stance.
2) Can you speed up the testing process with challenge studies, where volunteers willingly get infected?
It's a great question and I applaud the people who ask it and I applaud those signing up to do it. I'm not sure I am a huge fan, for both practical and ethical reasons. The devil is in the details. A challenge study has to show us a vaccine can prevent not just infection but prevent disease. Otherwise, how do I know the dose in the challenge study is the right dose? If you take 100 young people, 90 of them will get mild or no disease. Ten may end up in hospital and one in the ICU.
Also, the timeline. Can it let you skip Phase II of large efficacy trial? The reality for us is that we are about to start Phase II anyway. It would be months before a challenge trial could be designed. And ethically: everybody agrees there is a risk that is not zero of having very serious disease. To justify the risk, we have to be sure the benefit is worth it - that it actually shrunk the timeline. To just give us another data point, I find it hard to accept.
This technology allows us to scale up manufacturing and production.
3) What was seen preclinically in the animal models with Moderna's mRNA vaccines?
We have taken vaccines using our technology against eight different viruses, including two flu strains. In every case, in the preclinical model, we showed we could prevent disease, and when we got to antibody levels, we got the data we wanted to see. In doses of 25-100 micrograms, that usually ends up being a sweet spot where we see an effect. It's a good place as to the expectation of what we will see in Phase I trials.
4) Why is Moderna pursuing an mRNA virus instead of a traditional inactivated virus or recombinant one? This is an untried technology.
First, speed matters in a pandemic. If you have tech that can move much quicker, that makes a difference. The reason we have broken world records is that we have invested time and effort to be ready. We're starting from a platform where it's all based on synthetic biology.
Second, it's fundamental biology - we do not need to make an elaborate vaccine or stick a new virus in an old virus, or try to make a neutralizing but not binding virus. Our technology is basically mimicking the virus. All life works on making proteins through RNA. We have a biological advantage by teaching the immune system to do the right thing.
Third, this technology allows us to scale up manufacturing and production. We as a company have always seen this ahead of us. We invested in our own manufacturing facility two years ago. We have already envisioned scale up on two dimensions. Lot size and vaccines. Vaccines is the easier piece of it. If everybody gets 100 micrograms, it's not a heck of a lot. Prior to COVID, our lead program was a CMV (Cytomegalovirus) vaccine. We had envisioned launching Phase III next year. We had been already well on the path to scale up when COVID-19 caught us by surprise. This would be millions and millions of doses, but the train tracks have been laid.
5) People tend to think of vaccines as an on-off switch -- you get a vaccine and you're protected. But efficacy can be low or high (like the flu vs. measles vaccines). How good is good enough here for protection, and could we need several doses?
Probably around 50-60 percent efficacy is good enough for preventing a significant amount of disease and decreasing the R0. We will aim higher, but it's hard to estimate what degree of efficacy to prepare for until we do the trial. (For comparison, the average flu vaccine efficacy is around 50 percent.)
We anticipate a prime boost. If our immune system has never seen a virus, you can show you're getting to a certain antibody level and then remind the immune system (with another dose). A prime boost is optimal.
My only two competitors are the virus and the clock.
6) How would mutations affect a vaccine?
Coronaviruses tend to mutate the least compared to other viruses but it's entirely possible that it mutates. The report this week about those projected mutations on the spike protein have not been predicted to alter the critical antibodies.
As we scale up manufacturing, the ability to plug in a new genetic sequence and get a new vaccine out there will be very rapid.
For flu vaccine, we don't prove efficacy every year. If we get to the same place with an mRNA vaccine, we will just change the sequence and come out with a new vaccine. The path to approval would be much faster if we leverage the totality of efficacy data like we do for flu.
7) Will there be more than one vaccine and how will they be made available?
I hope so, I don't know. The path to making these available will go through a public-private partnership. It's not your typical commercial way of deploying a vaccine. But my only two competitors are the virus and the clock. We need everybody to be successful.
Kira Peikoff was the editor-in-chief of Leaps.org from 2017 to 2021. As a journalist, her work has appeared in The New York Times, Newsweek, Nautilus, Popular Mechanics, The New York Academy of Sciences, and other outlets. She is also the author of four suspense novels that explore controversial issues arising from scientific innovation: Living Proof, No Time to Die, Die Again Tomorrow, and Mother Knows Best. Peikoff holds a B.A. in Journalism from New York University and an M.S. in Bioethics from Columbia University. She lives in New Jersey with her husband and two young sons. Follow her on Twitter @KiraPeikoff.
After spaceflight record, NASA looks to protect astronauts on even longer trips
NASA astronaut Frank Rubio floats by the International Space Station’s “window to the world.” Yesterday, he returned from the longest single spaceflight by a U.S. astronaut on record - over one year. Exploring deep space will require even longer missions.
At T-minus six seconds, the main engines of the Atlantis Space Shuttle ignited, rattling its capsule “like a skyscraper in an earthquake,” according to astronaut Tom Jones, describing the 1988 launch. As the rocket lifted off and accelerated to three times the force of Earth's gravity, “It felt as if two of my friends were standing on my chest and wouldn’t get off.” But when Atlantis reached orbit, the main engines cut off, and the astronauts were suddenly weightless.
Since 1961, NASA has sent hundreds of astronauts into space while working to making their voyages safer and smoother. Yet, challenges remain. Weightlessness may look amusing when watched from Earth, but it has myriad effects on cognition, movement and other functions. When missions to space stretch to six months or longer, microgravity can impact astronauts’ health and performance, making it more difficult to operate their spacecraft.
Yesterday, NASA astronaut Frank Rubio returned to Earth after over one year, the longest single spaceflight for a U.S. astronaut. But this is just the start; longer and more complex missions into deep space loom ahead, from returning to the moon in 2025 to eventually sending humans to Mars. To ensure that these missions succeed, NASA is increasing efforts to study the biological effects and prevent harm.
The dangers of microgravity are real
A NASA report published in 2016 details a long list of incidents and near-misses caused – at least partly – by space-induced changes in astronauts’ vision and coordination. These issues make it harder to move with precision and to judge distance and velocity.
According to the report, in 1997, a resupply ship collided with the Mir space station, possibly because a crew member bumped into the commander during the final docking maneuver. This mishap caused significant damage to the space station.
Returns to Earth suffered from problems, too. The same report notes that touchdown speeds during the first 100 space shuttle landings were “outside acceptable limits. The fastest landing on record – 224 knots (258 miles) per hour – was linked to the commander’s momentary spatial disorientation.” Earlier, each of the six Apollo crews that landed on the moon had difficulty recognizing moon landmarks and estimating distances. For example, Apollo 15 landed in an unplanned area, ultimately straddling the rim of a five-foot deep crater on the moon, harming one of its engines.
Spaceflight causes unique stresses on astronauts’ brains and central nervous systems. NASA is working to reduce these harmful effects.
NASA
Space messes up your brain
In space, astronauts face the challenges of microgravity, ionizing radiation, social isolation, high workloads, altered circadian rhythms, monotony, confined living quarters and a high-risk environment. Among these issues, microgravity is one of the most consequential in terms of physiological changes. It changes the brain’s structure and its functioning, which can hurt astronauts’ performance.
The brain shifts upwards within the skull, displacing the cerebrospinal fluid, which reduces the brain’s cushioning. Essentially, the brain becomes crowded inside the skull like a pair of too-tight shoes.
That’s partly because of how being in space alters blood flow. On Earth, gravity pulls our blood and other internal fluids toward our feet, but our circulatory valves ensure that the fluids are evenly distributed throughout the body. In space, there’s not enough gravity to pull the fluids down, and they shift up, says Rachael D. Seidler, a physiologist specializing in spaceflight at the University of Florida and principal investigator on many space-related studies. The head swells and legs appear thinner, causing what astronauts call “puffy face chicken legs.”
“The brain changes at the structural and functional level,” says Steven Jillings, equilibrium and aerospace researcher at the University of Antwerp in Belgium. “The brain shifts upwards within the skull,” displacing the cerebrospinal fluid, which reduces the brain’s cushioning. Essentially, the brain becomes crowded inside the skull like a pair of too-tight shoes. Some of the displaced cerebrospinal fluid goes into cavities within the brain, called ventricles, enlarging them. “The remaining fluids pool near the chest and heart,” explains Jillings. After 12 consecutive months in space, one astronaut had a ventricle that was 25 percent larger than before the mission.
Some changes reverse themselves while others persist for a while. An example of a longer-lasting problem is spaceflight-induced neuro-ocular syndrome, which results in near-sightedness and pressure inside the skull. A study of approximately 300 astronauts shows near-sightedness affects about 60 percent of astronauts after long missions on the International Space Station (ISS) and more than 25 percent after spaceflights of only a few weeks.
Another long-term change could be the decreased ability of cerebrospinal fluid to clear waste products from the brain, Seidler says. That’s because compressing the brain also compresses its waste-removing glymphatic pathways, resulting in inflammation, vulnerability to injuries and worsening its overall health.
The effects of long space missions were best demonstrated on astronaut twins Scott and Mark Kelly. This NASA Twins Study showed multiple, perhaps permanent, changes in Scott after his 340-day mission aboard the ISS, compared to Mark, who remained on Earth. The differences included declines in Scott’s speed, accuracy and cognitive abilities that persisted longer than six months after returning to Earth in March 2016.
By the end of 2020, Scott’s cognitive abilities improved, but structural and physiological changes to his eyes still remained, he said in a BBC interview.
“It seems clear that the upward shift of the brain and compression of the surrounding tissues with ventricular expansion might not be a good thing,” Seidler says. “But, at this point, the long-term consequences to brain health and human performance are not really known.”
NASA astronaut Kate Rubins conducts a session for the Neuromapping investigation.
NASA
Staying sharp in space
To investigate how prolonged space travel affects the brain, NASA launched a new initiative called the Complement of Integrated Protocols for Human Exploration Research (CIPHER). “CIPHER investigates how long-duration spaceflight affects both brain structure and function,” says neurobehavioral scientist Mathias Basner at the University of Pennsylvania, a principal investigator for several NASA studies. “Through it, we can find out how the brain adapts to the spaceflight environment and how certain brain regions (behave) differently after – relative to before – the mission.”
To do this, he says, “Astronauts will perform NASA’s cognition test battery before, during and after six- to 12-month missions, and will also perform the same test battery in an MRI scanner before and after the mission. We have to make sure we better understand the functional consequences of spaceflight on the human brain before we can send humans safely to the moon and, especially, to Mars.”
As we go deeper into space, astronauts cognitive and physical functions will be even more important. “A trip to Mars will take about one year…and will introduce long communication delays,” Seidler says. “If you are on that mission and have a problem, it may take eight to 10 minutes for your message to reach mission control, and another eight to 10 minutes for the response to get back to you.” In an emergency situation, that may be too late for the response to matter.
“On a mission to Mars, astronauts will be exposed to stressors for unprecedented amounts of time,” Basner says. To counter them, NASA is considering the continuous use of artificial gravity during the journey, and Seidler is studying whether artificial gravity can reduce the harmful effects of microgravity. Some scientists are looking at precision brain stimulation as a way to improve memory and reduce anxiety due to prolonged exposure to radiation in space.
Other scientists are exploring how to protect neural stem cells (which create brain cells) from radiation damage, developing drugs to repair damaged brain cells and protect cells from radiation.
To boldly go where no astronauts have gone before, they must have optimal reflexes, vision and decision-making. In the era of deep space exploration, the brain—without a doubt—is the final frontier.
Additionally, NASA is scrutinizing each aspect of the mission, including astronaut exercise, nutrition and intellectual engagement. “We need to give astronauts meaningful work. We need to stimulate their sensory, cognitive and other systems appropriately,” Basner says, especially given their extreme confinement and isolation. The scientific experiments performed on the ISS – like studying how microgravity affects the ability of tissue to regenerate is a good example.
“We need to keep them engaged socially, too,” he continues. The ISS crew, for example, regularly broadcasts from space and answers prerecorded questions from students on Earth, and can engage with social media in real time. And, despite tight quarters, NASA is ensuring the crew capsule and living quarters on the moon or Mars include private space, which is critical for good mental health.
Exploring deep space builds on a foundation that began when astronauts first left the planet. With each mission, scientists learn more about spaceflight effects on astronauts’ bodies. NASA will be using these lessons to succeed with its plans to build science stations on the moon and, eventually, Mars.
“Through internally and externally led research, investigations implemented in space and in spaceflight simulations on Earth, we are striving to reduce the likelihood and potential impacts of neurostructural changes in future, extended spaceflight,” summarizes NASA scientist Alexandra Whitmire. To boldly go where no astronauts have gone before, they must have optimal reflexes, vision and decision-making. In the era of deep space exploration, the brain—without a doubt—is the final frontier.
A newly discovered brain cell may lead to better treatments for cognitive disorders
Swiss researchers have found a type of brain cell that appears to be a hybrid of the two other main types — and it could lead to new treatments for brain disorders.
Swiss researchers have discovered a third type of brain cell that appears to be a hybrid of the two other primary types — and it could lead to new treatments for many brain disorders.
The challenge: Most of the cells in the brain are either neurons or glial cells. While neurons use electrical and chemical signals to send messages to one another across small gaps called synapses, glial cells exist to support and protect neurons.
Astrocytes are a type of glial cell found near synapses. This close proximity to the place where brain signals are sent and received has led researchers to suspect that astrocytes might play an active role in the transmission of information inside the brain — a.k.a. “neurotransmission” — but no one has been able to prove the theory.
A new brain cell: Researchers at the Wyss Center for Bio and Neuroengineering and the University of Lausanne believe they’ve definitively proven that some astrocytes do actively participate in neurotransmission, making them a sort of hybrid of neurons and glial cells.
According to the researchers, this third type of brain cell, which they call a “glutamatergic astrocyte,” could offer a way to treat Alzheimer’s, Parkinson’s, and other disorders of the nervous system.
“Its discovery opens up immense research prospects,” said study co-director Andrea Volterra.
The study: Neurotransmission starts with a neuron releasing a chemical called a neurotransmitter, so the first thing the researchers did in their study was look at whether astrocytes can release the main neurotransmitter used by neurons: glutamate.
By analyzing astrocytes taken from the brains of mice, they discovered that certain astrocytes in the brain’s hippocampus did include the “molecular machinery” needed to excrete glutamate. They found evidence of the same machinery when they looked at datasets of human glial cells.
Finally, to demonstrate that these hybrid cells are actually playing a role in brain signaling, the researchers suppressed their ability to secrete glutamate in the brains of mice. This caused the rodents to experience memory problems.
“Our next studies will explore the potential protective role of this type of cell against memory impairment in Alzheimer’s disease, as well as its role in other regions and pathologies than those explored here,” said Andrea Volterra, University of Lausanne.
But why? The researchers aren’t sure why the brain needs glutamatergic astrocytes when it already has neurons, but Volterra suspects the hybrid brain cells may help with the distribution of signals — a single astrocyte can be in contact with thousands of synapses.
“Often, we have neuronal information that needs to spread to larger ensembles, and neurons are not very good for the coordination of this,” researcher Ludovic Telley told New Scientist.
Looking ahead: More research is needed to see how the new brain cell functions in people, but the discovery that it plays a role in memory in mice suggests it might be a worthwhile target for Alzheimer’s disease treatments.
The researchers also found evidence during their study that the cell might play a role in brain circuits linked to seizures and voluntary movements, meaning it’s also a new lead in the hunt for better epilepsy and Parkinson’s treatments.
“Our next studies will explore the potential protective role of this type of cell against memory impairment in Alzheimer’s disease, as well as its role in other regions and pathologies than those explored here,” said Volterra.