Antibody Testing Alone is Not the Key to Re-Opening Society
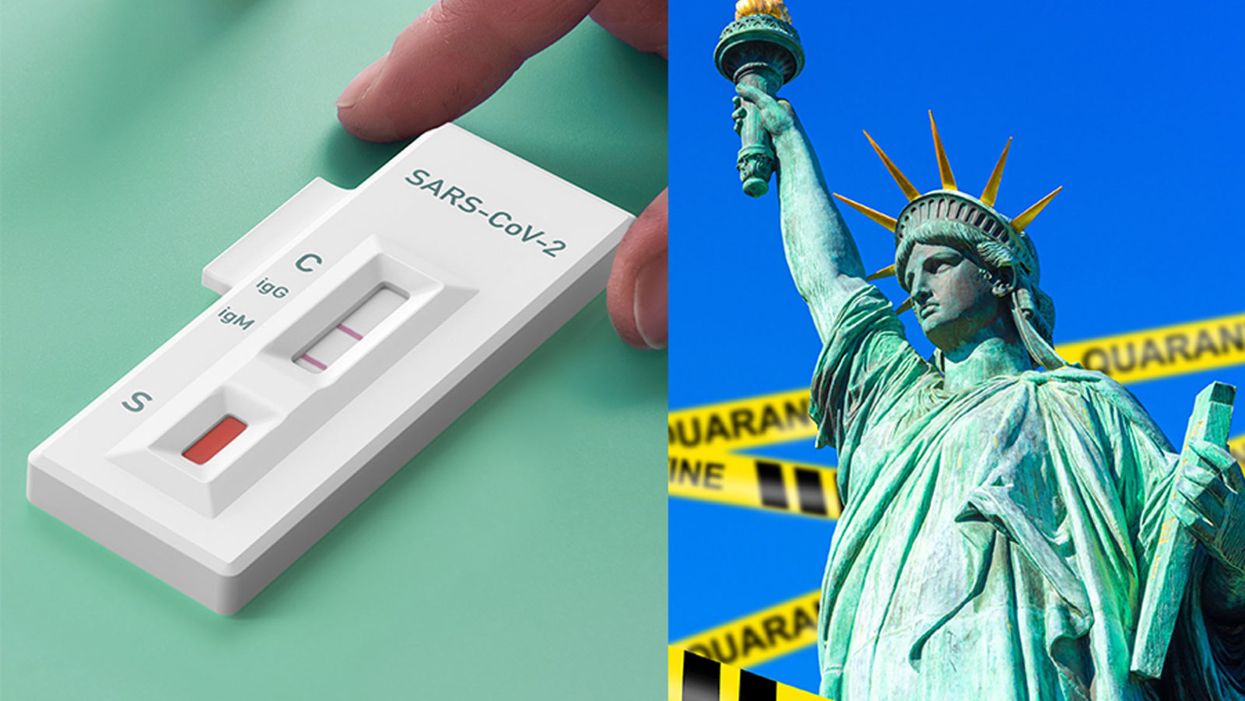
Immunity tests have too many unknowns right now to make them very useful in determining protective antibody status.
[Editor's Note: We asked experts from different specialties to weigh in on a timely Big Question: "How should immunity testing play a role in re-opening society?" Below, a virologist offers her perspective.]
With the advent of serology testing and increased emphasis on "re-opening" America, public health officials have begun considering whether or not people who have recovered from COVID-19 can safely re-enter the workplace.
"Immunity certificates cannot certify what is not known."
Conventional wisdom holds that people who have developed antibodies in response to infection with SARS-CoV-2, the coronavirus that causes COVID-19, are likely to be immune to reinfection.
For most acute viral infections, this is generally true. However, SARS-CoV-2 is a new pathogen, and there are currently many unanswered questions about immunity. Can recovered patients be reinfected or transmit the virus? Does symptom severity determine how protective responses will be after recovery? How long will protection last? Understanding these basic features is essential to phased re-opening of the government and economy for people who have recovered from COVID-19.
One mechanism that has been considered is issuing "immunity certificates" to individuals with antibodies against SARS-CoV-2. These certificates would verify that individuals have already recovered from COVID-19, and thus have antibodies in their blood that will protect them against reinfection, enabling them to safely return to work and participate in society. Although this sounds reasonable in theory, there are many practical reasons why this is not a wise policy decision to ease off restrictive stay-home orders and distancing practices.
Too Many Scientific Unknowns
Serology tests measure antibodies in the serum—the liquid component of blood, which is where the antibodies are located. In this case, serology tests measure antibodies that specifically bind to SARS-CoV-2 virus particles. Usually when a person is infected with a virus, they develop antibodies that can "recognize" that virus, so the presence of SARS-CoV-2 antibodies indicates that a person has been previously exposed to the virus. Broad serology testing is critical to knowing how many people have been infected with SARS-CoV-2, since testing capacity for the virus itself has been so low.
Tests for the virus measure amounts of SARS-CoV-2 RNA—the virus's genetic material—directly, and thus will not detect the virus once a person has recovered. Thus, the majority of people who were not severely ill and did not require hospitalization, or did not have direct contact with a confirmed case, will not test positive for the virus weeks after they have recovered and can only determine if they had COVID-19 by testing for antibodies.
In most cases, for most pathogens, antibodies are also neutralizing, meaning they bind to the virus and render it incapable of infecting cells, and this protects against future infections. Immunity certificates are based on the assumption that people with antibodies specific for SARS-CoV-2 will be protected against reinfection. The problem is that we've only known that SARS-CoV-2 existed for a little over four months. Although studies so far indicate that most (but not all) patients with confirmed COVID-19 cases develop antibodies, we don't know the extent to which antibodies are protective against reinfection, or how long that protection will last. Immunity certificates cannot certify what is not known.
The limited data so far is encouraging with regard to protective immunity. Most of the patient sera tested for antibodies show reasonable titers of IgG, the type of antibodies most likely to be neutralizing. Furthermore, studies have shown that these IgG antibodies are capable of neutralizing surrogate viruses as well as infectious SARS-CoV-2 in laboratory tests. In addition, rhesus monkeys that were experimentally infected with SARS-CoV-2 and allowed to recover were protected from reinfection after a subsequent experimental challenge. These data tentatively suggest that most people are likely to develop neutralizing IgG, and protective immunity, after being infected by SARS-CoV-2.
However, not all COVID-19 patients do produce high levels of antibodies specific for SARS-CoV-2. A small number of patients in one study had no detectable neutralizing IgG. There have also been reports of patients in South Korea testing PCR positive after a prior negative test, indicating reinfection or reactivation. These cases may be explained by the sensitivity of the PCR test, and no data have been produced to indicate that these cases are genuine reinfection or recurrence of viral infection.
Complicating matters further, not all serology tests measure antibody titers. Some rapid serology tests are designed to be binary—the test can either detect antibodies or not, but does not give information about the amount of antibodies circulating. Based on our current knowledge, we cannot be certain that merely having any level of detectable antibodies alone guarantees protection from reinfection, or from a subclinical reinfection that might not cause a second case of COVID-19, but could still result in transmission to others. These unknowns remain problematic even with tests that accurately detect the presence of antibodies—which is not a given today, as many of the newly available tests are reportedly unreliable.
A Logistical and Ethical Quagmire
While most people are eager to cast off the isolation of physical distancing and resume their normal lives, mere desire to return to normality is not an indicator of whether those antibodies actually work, and no certificate can confer immune protection. Furthermore, immunity certificates could lead to some complicated logistical and ethical issues. If antibodies do not guarantee protective immunity, certifying that they do could give antibody-positive people a false sense of security, causing them to relax infection control practices such as distancing and hand hygiene.
"We should not, however, place our faith in assumptions and make return to normality contingent on an arbitrary and uninformative piece of paper."
Certificates could be forged, putting susceptible people at higher exposure risk. It's not clear who would issue them, what they would entitle the bearer to do or not do, or how certification would be verified or enforced. There are many ways in which such certificates could be used as a pretext to discriminate against people based on health status, in addition to disability, race, and socioeconomic status. Tracking people based on immune status raises further concerns about privacy and civil rights.
Rather than issuing documents confirming immune status, we should instead "re-open" society cautiously, with widespread virus and serology testing to accurately identify and isolate infected cases rapidly, with immediate contact tracing to safely quarantine and monitor those at exposure risk. Broad serosurveillance must be coupled with functional assays for neutralization activity to begin assessing how protective antibodies might actually be against SARS-CoV-2 infection. To understand how long immunity lasts, we should study antibodies, as well as the functional capabilities of other components of the larger immune system, such as T cells, in recovered COVID-19 patients over time.
We should not, however, place our faith in assumptions and make return to normality contingent on an arbitrary and uninformative piece of paper. Re-opening society, the government, and the economy depends not only on accurately determining how many people have antibodies to SARS-CoV-2, but on a deeper understanding of how those antibodies work to provide protection.
Can a Non-Invasive Magnetic Helmet Treat Brain Cancer?
Glioblastoma is an aggressive and deadly brain cancer, causing more than 10,000 deaths in the US per year. In the last 30 years there has only been limited improvement in the survival rate despite advances in radiation therapy and chemotherapy. Today the typical survival rate is just 14 months and that extra time is spent suffering from the adverse and often brutal effects of radiation and chemotherapy.
Scientists are trying to design more effective treatments for glioblastoma with fewer side effects, and a team at the Department of Neurosurgery at Houston Methodist Hospital has created a magnetic helmet-based treatment called oncomagnetic therapy: a promising non-invasive treatment for shrinking cancerous tumors. In the first patient tried, the device was able to reduce the tumor of a glioblastoma patient by 31%. The researchers caution, however, that much more research is needed to determine its safety and effectiveness.
How It Works
“The whole idea originally came from a conversation I had with General Norman Schwarzkopf, a supposedly brilliant military strategist,” says David Baskin, professor of neurosurgery and leader of the effort at Houston Methodist. “I asked him what is the secret to your success and he said, ‘Energy. Take out the power grid and the enemy can't communicate.’ So I thought about what supplies [energy to] cancer, especially brain cancer.”
Baskin came up with the idea of targeting the mitochondria, which process and produce energy for cancer cells.
"This is the most exciting thing in glioblastoma treatment I've seen since I've been a neurosurgeon, but it is very preliminary,” Baskin says.
The magnetic helmet creates a powerful oscillating magnetic field. At a set range of frequencies and timings, it disrupts the flow of electrons in the mitochondria of cancer cells. This leads to a release of certain chemicals called Reactive Oxygen Species, or ROS. In normal cells, this excess ROS is much lower, and it's neutralized by other chemicals called antioxidants.
However, cancer cells already have more ROS: they grow rapidly and uncontrollably, so their mitochondria need to produce more energy which in turn generates more ROS. By using the powerful magnetic field, levels of ROS get so high that the malignant cells are torn apart.
The biggest challenge was working out the specific range of frequencies and timing parameters they needed to use to kill cancer cells. It took skill, intuition, luck and lots of experiments. The helmet could theoretically be used to treat all types of glioblastoma.
Developing the magnetic helmet was a collaborative process. Santosh Helekar is a neuroscientist at Houston Methodist Research Institute and the director of oncomagnetics (magnetic cancer therapies) at the Peak Center in Houston Methodist Hospital. His previous invention with colleagues gave the team a starting point to build on. “About 7 years back I developed a portable brain magnetic stimulation device to conduct brain research,” Helekar says. “We [then] conducted a pilot clinical trial in stroke patients. The results were promising.”
Helekar presented his findings to neurosurgeons including Baskin. They decided to collaborate. With a team of scientists behind them, they modified the device to kill cancer cells.
The magnetic helmet studied for treatment of glioblastoma
Dr. David Baskin
Initial Results
After success in the lab, the team got FDA approval to conduct a compassionate trial in a 53-year-old man with end-stage glioblastoma. He had tried every other treatment available. But within 30 days of using the magnetic helmet his tumor shrank by 31%.
Sadly, 36 days into the treatment, the patient had an unrelated head injury due to a fall. The treatment was paused and he later died of the injury. Autopsy results of his brain highlighted the dramatic reduction in tumor cells.
Baskin says, “This is the most exciting thing in glioblastoma treatment I've seen since I've been a neurosurgeon, but it is very preliminary.”
The helmet is part of a growing number of non-invasive cancer treatments. One device that is currently being used by glioblastoma patients is Optune. It uses electric fields called tumor treating fields to slow down cell division and has been through a successful phase 3 clinical trial.
The magnetic helmet has the promise to be another useful non-invasive treatment according to Professor Gabriel Zada, a neurosurgeon and director of the USC Brain Tumor Center. “We're learning that various electromagnetic fields and tumor treating fields appear to play a role in glioblastoma. So there is some precedent for this though the tumor treating fields work a little differently. I think there is major potential for it to be effective but of course it will require some trials.”
Professor Jonathan Sherman, a neurosurgeon and director of neuro-oncology at West Virginia University, reiterates the need for further testing. “It sounds interesting but it’s too early to tell what kind of long-term efficacy you get. We do not have enough data. Also if you’re disrupting [the magnetic field] you could negatively impact a patient. You could be affecting the normal conduction of electromagnetic activity in the brain.”
The team is currently extending their research. They are now testing the treatment in two other patients with end-stage glioblastoma. The immediate challenge is getting FDA approval for those at an earlier stage of the disease who are more likely to benefit.
The Future
Baskin and the team are designing a clinical trial in the U.S., .U.K. and Germany. After positive results in cell cultures, they’re in negotiations to collaborate with other researchers in using the technology for lung and breast cancer. With breast cancer, the soft tissue is easier to access so a magnetic device could be worn over the breast.
“My hope is to develop a treatment to treat and hopefully cure glioblastoma without radiation or chemotherapy,” Baskin says. “We're onto a strategy that could make a huge difference for patients with this disease and probably for patients with many other forms of cancer.”
Astronaut and Expedition 64 Flight Engineer Soichi Noguchi of the Japan Aerospace Exploration Agency displays Extra Dwarf Pak Choi plants growing aboard the International Space Station. The plants were grown for the Veggie study which is exploring space agriculture as a way to sustain astronauts on future missions to the Moon or Mars.
Astronauts at the International Space Station today depend on pre-packaged, freeze-dried food, plus some fresh produce thanks to regular resupply missions. This supply chain, however, will not be available on trips further out, such as the moon or Mars. So what are astronauts on long missions going to eat?
Going by the options available now, says Christel Paille, an engineer at the European Space Agency, a lunar expedition is likely to have only dehydrated foods. “So no more fresh product, and a limited amount of already hydrated product in cans.”
For the Mars mission, the situation is a bit more complex, she says. Prepackaged food could still constitute most of their food, “but combined with [on site] production of certain food products…to get them fresh.” A Mars mission isn’t right around the corner, but scientists are currently working on solutions for how to feed those astronauts. A number of boundary-pushing efforts are now underway.
The logistics of growing plants in space, of course, are very different from Earth. There is no gravity, sunlight, or atmosphere. High levels of ionizing radiation stunt plant growth. Plus, plants take up a lot of space, something that is, ironically, at a premium up there. These and special nutritional requirements of spacefarers have given scientists some specific and challenging problems.
To study fresh food production systems, NASA runs the Vegetable Production System (Veggie) on the ISS. Deployed in 2014, Veggie has been growing salad-type plants on “plant pillows” filled with growth media, including a special clay and controlled-release fertilizer, and a passive wicking watering system. They have had some success growing leafy greens and even flowers.
"Ideally, we would like a system which has zero waste and, therefore, needs zero input, zero additional resources."
A larger farming facility run by NASA on the ISS is the Advanced Plant Habitat to study how plants grow in space. This fully-automated, closed-loop system has an environmentally controlled growth chamber and is equipped with sensors that relay real-time information about temperature, oxygen content, and moisture levels back to the ground team at Kennedy Space Center in Florida. In December 2020, the ISS crew feasted on radishes grown in the APH.
“But salad doesn’t give you any calories,” says Erik Seedhouse, a researcher at the Applied Aviation Sciences Department at Embry-Riddle Aeronautical University in Florida. “It gives you some minerals, but it doesn’t give you a lot of carbohydrates.” Seedhouse also noted in his 2020 book Life Support Systems for Humans in Space: “Integrating the growing of plants into a life support system is a fiendishly difficult enterprise.” As a case point, he referred to the ESA’s Micro-Ecological Life Support System Alternative (MELiSSA) program that has been running since 1989 to integrate growing of plants in a closed life support system such as a spacecraft.
Paille, one of the scientists running MELiSSA, says that the system aims to recycle the metabolic waste produced by crew members back into the metabolic resources required by them: “The aim is…to come [up with] a closed, sustainable system which does not [need] any logistics resupply.” MELiSSA uses microorganisms to process human excretions in order to harvest carbon dioxide and nitrate to grow plants. “Ideally, we would like a system which has zero waste and, therefore, needs zero input, zero additional resources,” Paille adds.
Microorganisms play a big role as “fuel” in food production in extreme places, including in space. Last year, researchers discovered Methylobacterium strains on the ISS, including some never-seen-before species. Kasthuri Venkateswaran of NASA’s Jet Propulsion Laboratory, one of the researchers involved in the study, says, “[The] isolation of novel microbes that help to promote the plant growth under stressful conditions is very essential… Certain bacteria can decompose complex matter into a simple nutrient [that] the plants can absorb.” These microbes, which have already adapted to space conditions—such as the absence of gravity and increased radiation—boost various plant growth processes and help withstand the harsh physical environment.
MELiSSA, says Paille, has demonstrated that it is possible to grow plants in space. “This is important information because…we didn’t know whether the space environment was affecting the biological cycle of the plant…[and of] cyanobacteria.” With the scientific and engineering aspects of a closed, self-sustaining life support system becoming clearer, she says, the next stage is to find out if it works in space. They plan to run tests recycling human urine into useful components, including those that promote plant growth.
The MELiSSA pilot plant uses rats currently, and needs to be translated for human subjects for further studies. “Demonstrating the process and well-being of a rat in terms of providing water, sufficient oxygen, and recycling sufficient carbon dioxide, in a non-stressful manner, is one thing,” Paille says, “but then, having a human in the loop [means] you also need to integrate user interfaces from the operational point of view.”
Growing food in space comes with an additional caveat that underscores its high stakes. Barbara Demmig-Adams from the Department of Ecology and Evolutionary Biology at the University of Colorado Boulder explains, “There are conditions that actually will hurt your health more than just living here on earth. And so the need for nutritious food and micronutrients is even greater for an astronaut than for [you and] me.”
Demmig-Adams, who has worked on increasing the nutritional quality of plants for long-duration spaceflight missions, also adds that there is no need to reinvent the wheel. Her work has focused on duckweed, a rather unappealingly named aquatic plant. “It is 100 percent edible, grows very fast, it’s very small, and like some other floating aquatic plants, also produces a lot of protein,” she says. “And here on Earth, studies have shown that the amount of protein you get from the same area of these floating aquatic plants is 20 times higher compared to soybeans.”
Aquatic plants also tend to grow well in microgravity: “Plants that float on water, they don’t respond to gravity, they just hug the water film… They don’t need to know what’s up and what’s down.” On top of that, she adds, “They also produce higher concentrations of really important micronutrients, antioxidants that humans need, especially under space radiation.” In fact, duckweed, when subjected to high amounts of radiation, makes nutrients called carotenoids that are crucial for fighting radiation damage. “We’ve looked at dozens and dozens of plants, and the duckweed makes more of this radiation fighter…than anything I’ve seen before.”
Despite all the scientific advances and promising leads, no one really knows what the conditions so far out in space will be and what new challenges they will bring. As Paille says, “There are known unknowns and unknown unknowns.”
One definite “known” for astronauts is that growing their food is the ideal scenario for space travel in the long term since “[taking] all your food along with you, for best part of two years, that’s a lot of space and a lot of weight,” as Seedhouse says. That said, once they land on Mars, they’d have to think about what to eat all over again. “Then you probably want to start building a greenhouse and growing food there [as well],” he adds.
And that is a whole different challenge altogether.