Food Poisoning Outbreaks Are Still A Problem. Powerful Tech Is Fighting Back.
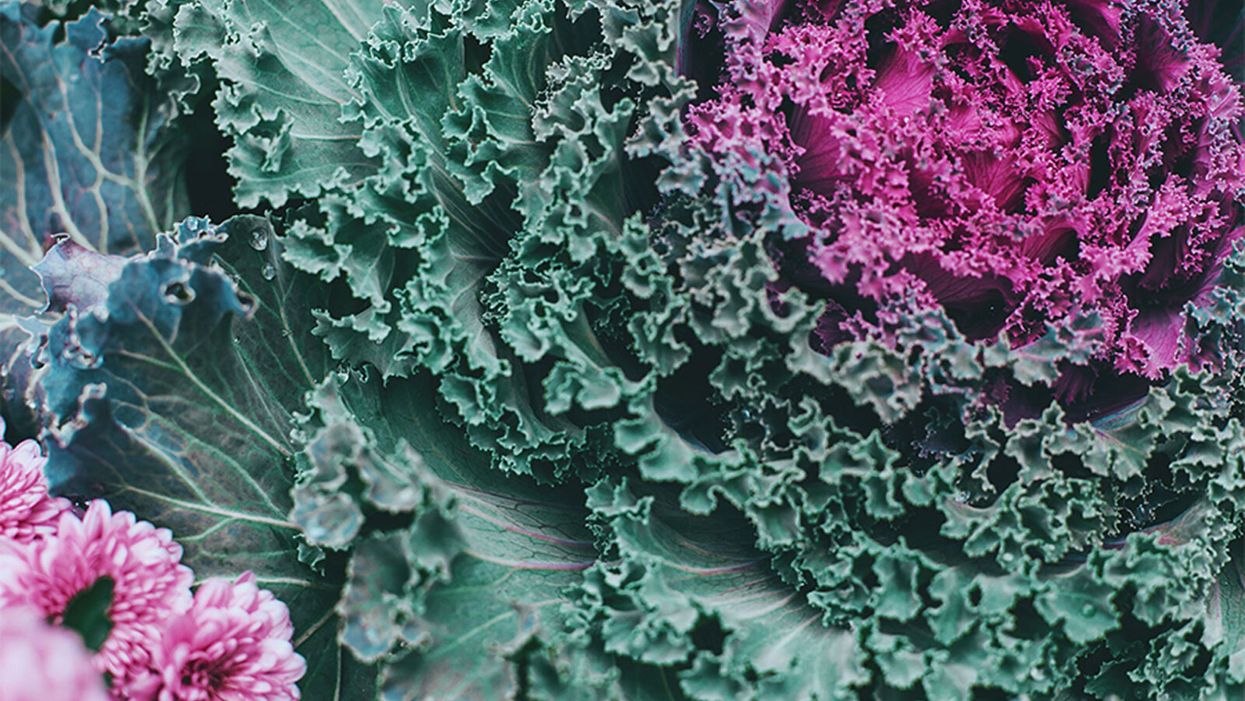
The latest tools, like whole genome sequencing, are allowing food outbreaks to be identified and stopped more quickly.
With the pandemic at the forefront of everyone's minds, many people have wondered if food could be a source of coronavirus transmission. Luckily, that "seems unlikely," according to the CDC, but foodborne illnesses do still sicken a whopping 48 million people per year.
Whole genome sequencing is like "going from an eight-bit image—maybe like what you would see in Minecraft—to a high definition image."
In normal times, when there isn't a historic global health crisis infecting millions and affecting the lives of billions, foodborne outbreaks are real and frightening, potentially deadly, and can cause widespread fear of particular foods. Think of Romaine lettuce spreading E. coli last year— an outbreak that infected more than 500 people and killed eight—or peanut butter spreading salmonella in 2008, which infected 167 people.
The technologies available to detect and prevent the next foodborne disease outbreak have improved greatly over the past 30-plus years, particularly during the past decade, and better, more nimble technologies are being developed, according to experts in government, academia, and private industry. The key to advancing detection of harmful foodborne pathogens, they say, is increasing speed and portability of detection, and the precision of that detection.
Getting to Rapid Results
Researchers at Purdue University have recently developed a lateral flow assay that, with the help of a laser, can detect toxins and pathogenic E. coli. Lateral flow assays are cheap and easy to use; a good example is a home pregnancy test. You place a liquid or liquefied sample on a piece of paper designed to detect a single substance and soon after you get the results in the form of a colored line: yes or no.
"They're a great portable tool for us for food contaminant detection," says Carmen Gondhalekar, a fifth-year biomedical engineering graduate student at Purdue. "But one of the areas where paper-based lateral flow assays could use improvement is in multiplexing capability and their sensitivity."
J. Paul Robinson, a professor in Purdue's Colleges of Veterinary Medicine and Engineering, and Gondhalekar's advisor, agrees. "One of the fundamental problems that we have in detection is that it is hard to identify pathogens in complex samples," he says.
When it comes to foodborne disease outbreaks, you don't always know what substance you're looking for, so an assay made to detect only a single substance isn't always effective. The goal of the project at Purdue is to make assays that can detect multiple substances at once.
These assays would be more complex than a pregnancy test. As detailed in Gondhalekar's recent paper, a laser pulse helps create a spectral signal from the sample on the assay paper, and the spectral signal is then used to determine if any unique wavelengths associated with one of several toxins or pathogens are present in the sample. Though the handheld technology has yet to be built, the idea is that the results would be given on the spot. So someone in the field trying to track the source of a Salmonella infection could, for instance, put a suspected lettuce sample on the assay and see if it has the pathogen on it.
"What our technology is designed to do is to give you a rapid assessment of the sample," says Robinson. "The goal here is speed."
Seeing the Pathogen in "High-Def"
"One in six Americans will get a foodborne illness every year," according to Dr. Heather Carleton, a microbiologist at the Centers for Disease Control and Prevention's Enteric Diseases Laboratory Branch. But not every foodborne outbreak makes the news. In 2017 alone, the CDC monitored between 18 and 37 foodborne poison clusters per week and investigated 200 multi-state clusters. Hardboiled eggs, ground beef, chopped salad kits, raw oysters, frozen tuna, and pre-cut melon are just a taste of the foods that were investigated last year for different strains of listeria, salmonella, and E. coli.
At the heart of the CDC investigations is PulseNet, a national network of laboratories that uses DNA fingerprinting to detect outbreaks at local and regional levels. This is how it works: When a patient gets sick—with symptoms like vomiting and fever, for instance—they will go to a hospital or clinic for treatment. Since we're talking about foodborne illnesses, a clinician will likely take a stool sample from the patient and send it off to a laboratory to see if there is a foodborne pathogen, like salmonella, E. Coli, or another one. If it does contain a potentially harmful pathogen, then a bacterial isolate of that identified sample is sent to a regional public health lab so that whole genome sequencing can be performed.
Whole genome sequencing can differentiate "virtually all" strains of foodborne pathogens, no matter the species, according to the FDA.
Whole genome sequencing is a method for reading the entire genome of a bacterial isolate (or from any organism, for that matter). Instead of working with a couple dozen data points, now you're working with millions of base pairs. Carleton likes to describe it as "going from an eight-bit image—maybe like what you would see in Minecraft—to a high definition image," she says. "It's really an evolution of how we detect foodborne illnesses and identify outbreaks."
If the bacterial isolate matches another in the CDC's database, this means there could be a potential outbreak and an investigation may be started, with the goal of tracking the pathogen to its source.
Whole genome sequencing has been a relatively recent shift in foodborne disease detection. For more than 20 years, the standard technique for analyzing pathogens in foodborne disease outbreaks was pulsed-field gel electrophoresis. This method creates a DNA fingerprint for each sample in the form of a pattern of about 15-30 "bands," with each band representing a piece of DNA. Researchers like Carleton can use this fingerprint to see if two samples are from the same bacteria. The problem is that 15-30 bands are not enough to differentiate all isolates. Some isolates whose bands look very similar may actually come from different sources and some whose bands look different may be from the same source. But if you can see the entire DNA fingerprint, then you don't have that issue. That's where whole genome sequencing comes in.
Although the PulseNet team had piloted whole genome sequencing as early as 2013, it wasn't until July of last year that the transition to using whole genome sequencing for all pathogens was complete. Though whole genome sequencing requires far more computing power to generate, analyze, and compare those millions of data points, the payoff is huge.
Stopping Outbreaks Sooner
The U.S. Food and Drug Administration (FDA) acquired their first whole genome sequencers in 2008, according to Dr. Eric Brown, the Director of the Division of Microbiology in the FDA's Office of Regulatory Science. Since then, through their GenomeTrakr program, a network of more than 60 domestic and international labs, the FDA has sequenced and publicly shared more than 400,000 isolates. "The impact of what whole genome sequencing could do to resolve a foodborne outbreak event was no less impactful than when NASA turned on the Hubble Telescope for the first time," says Brown.
Whole genome sequencing has helped identify strains of Salmonella that prior methods were unable to differentiate. In fact, whole genome sequencing can differentiate "virtually all" strains of foodborne pathogens, no matter the species, according to the FDA. This means it takes fewer clinical cases—fewer sick people—to detect and end an outbreak.
And perhaps the largest benefit of whole genome sequencing is that these detailed sequences—the millions of base pairs—can imply geographic location. The genomic information of bacterial strains can be different depending on the area of the country, helping these public health agencies eventually track the source of outbreaks—a restaurant, a farm, a food-processing center.
Coming Soon: "Lab in a Backpack"
Now that whole genome sequencing has become the go-to technology of choice for analyzing foodborne pathogens, the next step is making the process nimbler and more portable. Putting "the lab in a backpack," as Brown says.
The CDC's Carleton agrees. "Right now, the sequencer we use is a fairly big box that weighs about 60 pounds," she says. "We can't take it into the field."
A company called Oxford Nanopore Technologies is developing handheld sequencers. Their devices are meant to "enable the sequencing of anything by anyone anywhere," according to Dan Turner, the VP of Applications at Oxford Nanopore.
"The sooner that we can see linkages…the sooner the FDA gets in action to mitigate the problem and put in some kind of preventative control."
"Right now, sequencing is very much something that is done by people in white coats in laboratories that are set up for that purpose," says Turner. Oxford Nanopore would like to create a new, democratized paradigm.
The FDA is currently testing these types of portable sequencers. "We're very excited about it. We've done some pilots, to be able to do that sequencing in the field. To actually do it at a pond, at a river, at a canal. To do it on site right there," says Brown. "This, of course, is huge because it means we can have real-time sequencing capability to stay in step with an actual laboratory investigation in the field."
"The timeliness of this information is critical," says Marc Allard, a senior biomedical research officer and Brown's colleague at the FDA. "The sooner that we can see linkages…the sooner the FDA gets in action to mitigate the problem and put in some kind of preventative control."
At the moment, the world is rightly focused on COVID-19. But as the danger of one virus subsides, it's only a matter of time before another pathogen strikes. Hopefully, with new and advancing technology like whole genome sequencing, we can stop the next deadly outbreak before it really gets going.
Stronger psychedelics that rewire the brain, with Doug Drysdale
Today's podcast episode features Doug Drysdale, CEO of Cybin, a company that is leading innovations in psilocybin, mushrooms that may help people with anxiety and depression.
A promising development in science in recent years has been the use technology to optimize something natural. One-upping nature's wisdom isn't easy. In many cases, we haven't - and maybe we can't - figure it out. But today's episode features a fascinating example: using tech to optimize psychedelic mushrooms.
Listen on Apple | Listen on Spotify | Listen on Stitcher | Listen on Amazon | Listen on Google
These mushrooms have been used for religious, spiritual and medicinal purposes for thousands of years, but only in the past several decades have scientists brought psychedelics into the lab to enhance them and maximize their therapeutic value.
Today’s podcast guest, Doug Drysdale, is doing important work to lead this effort. Drysdale is the CEO of a company called Cybin that has figured out how to make psilocybin more potent, so it can be administered in smaller doses without side effects.
The natural form of psilocybin has been studied increasingly in the realm of mental health. Taking doses of these mushrooms appears to help people with anxiety and depression by spurring the development of connections in the brain, an example of neuroplasticity. The process basically shifts the adult brain from being fairly rigid like dried clay into a malleable substance like warm wax - the state of change that's constantly underway in the developing brains of children.
Neuroplasticity in adults seems to unlock some of our default ways of of thinking, the habitual thought patterns that’ve been associated with various mental health problems. Some promising research suggests that psilocybin causes a reset of sorts. It makes way for new, healthier thought patterns.
So what is Drysdale’s secret weapon to bring even more therapeutic value to psilocybin? It’s a process called deuteration. It focuses on the hydrogen atoms in psilocybin. These atoms are very light and don’t stick very well to carbon, which is another atom in psilocybin. As a result, our bodies can easily breaks down the bonds between the hydrogen and carbon atoms. For many people, that means psilocybin gets cleared from the body too quickly, before it can have a therapeutic benefit.
In deuteration, scientists do something simple but ingenious: they replace the hydrogen atoms with a molecule called deuterium. It’s twice as heavy as hydrogen and forms tighter bonds with the carbon. Because these pairs are so rock-steady, they slow down the rate at which psilocybin is metabolized, so it has more sustained effects on our brains.
Cybin isn’t Drysdale’s first go around at this - far from it. He has over 30 years of experience in the healthcare sector. During this time he’s raised around $4 billion of both public and private capital, and has been named Ernst and Young Entrepreneur of the Year. Before Cybin, he was the founding CEO of a pharmaceutical company called Alvogen, leading it from inception to around $500 million in revenues, across 35 countries. Drysdale has also been the head of mergers and acquisitions at Actavis Group, leading 15 corporate acquisitions across three continents.
In this episode, Drysdale walks us through the promising research of his current company, Cybin, and the different therapies he’s developing for anxiety and depression based not just on psilocybin but another psychedelic compound found in plants called DMT. He explains how they seem to have such powerful effects on the brain, as well as the potential for psychedelics to eventually support other use cases, including helping us strive toward higher levels of well-being. He goes on to discuss his views on mindfulness and lifestyle factors - such as optimal nutrition - that could help bring out hte best in psychedelics.
Show links:
Doug Drysdale full bio
Doug Drysdale twitter
Cybin website
Cybin development pipeline
Cybin's promising phase 2 research on depression
Johns Hopkins psychedelics research and psilocybin research
Mets owner Steve Cohen invests in psychedelic therapies
Doug Drysdale, CEO of Cybin
How the body's immune resilience affects our health and lifespan
Immune cells battle an infection.
Story by Big Think
It is a mystery why humans manifest vast differences in lifespan, health, and susceptibility to infectious diseases. However, a team of international scientists has revealed that the capacity to resist or recover from infections and inflammation (a trait they call “immune resilience”) is one of the major contributors to these differences.
Immune resilience involves controlling inflammation and preserving or rapidly restoring immune activity at any age, explained Weijing He, a study co-author. He and his colleagues discovered that people with the highest level of immune resilience were more likely to live longer, resist infection and recurrence of skin cancer, and survive COVID and sepsis.
Measuring immune resilience
The researchers measured immune resilience in two ways. The first is based on the relative quantities of two types of immune cells, CD4+ T cells and CD8+ T cells. CD4+ T cells coordinate the immune system’s response to pathogens and are often used to measure immune health (with higher levels typically suggesting a stronger immune system). However, in 2021, the researchers found that a low level of CD8+ T cells (which are responsible for killing damaged or infected cells) is also an important indicator of immune health. In fact, patients with high levels of CD4+ T cells and low levels of CD8+ T cells during SARS-CoV-2 and HIV infection were the least likely to develop severe COVID and AIDS.
Individuals with optimal levels of immune resilience were more likely to live longer.
In the same 2021 study, the researchers identified a second measure of immune resilience that involves two gene expression signatures correlated with an infected person’s risk of death. One of the signatures was linked to a higher risk of death; it includes genes related to inflammation — an essential process for jumpstarting the immune system but one that can cause considerable damage if left unbridled. The other signature was linked to a greater chance of survival; it includes genes related to keeping inflammation in check. These genes help the immune system mount a balanced immune response during infection and taper down the response after the threat is gone. The researchers found that participants who expressed the optimal combination of genes lived longer.
Immune resilience and longevity
The researchers assessed levels of immune resilience in nearly 50,000 participants of different ages and with various types of challenges to their immune systems, including acute infections, chronic diseases, and cancers. Their evaluation demonstrated that individuals with optimal levels of immune resilience were more likely to live longer, resist HIV and influenza infections, resist recurrence of skin cancer after kidney transplant, survive COVID infection, and survive sepsis.
However, a person’s immune resilience fluctuates all the time. Study participants who had optimal immune resilience before common symptomatic viral infections like a cold or the flu experienced a shift in their gene expression to poor immune resilience within 48 hours of symptom onset. As these people recovered from their infection, many gradually returned to the more favorable gene expression levels they had before. However, nearly 30% who once had optimal immune resilience did not fully regain that survival-associated profile by the end of the cold and flu season, even though they had recovered from their illness.
Intriguingly, some people who are 90+ years old still have optimal immune resilience, suggesting that these individuals’ immune systems have an exceptional capacity to control inflammation and rapidly restore proper immune balance.
This could suggest that the recovery phase varies among people and diseases. For example, young female sex workers who had many clients and did not use condoms — and thus were repeatedly exposed to sexually transmitted pathogens — had very low immune resilience. However, most of the sex workers who began reducing their exposure to sexually transmitted pathogens by using condoms and decreasing their number of sex partners experienced an improvement in immune resilience over the next 10 years.
Immune resilience and aging
The researchers found that the proportion of people with optimal immune resilience tended to be highest among the young and lowest among the elderly. The researchers suggest that, as people age, they are exposed to increasingly more health conditions (acute infections, chronic diseases, cancers, etc.) which challenge their immune systems to undergo a “respond-and-recover” cycle. During the response phase, CD8+ T cells and inflammatory gene expression increase, and during the recovery phase, they go back down.
However, over a lifetime of repeated challenges, the immune system is slower to recover, altering a person’s immune resilience. Intriguingly, some people who are 90+ years old still have optimal immune resilience, suggesting that these individuals’ immune systems have an exceptional capacity to control inflammation and rapidly restore proper immune balance despite the many respond-and-recover cycles that their immune systems have faced.
Public health ramifications could be significant. Immune cell and gene expression profile assessments are relatively simple to conduct, and being able to determine a person’s immune resilience can help identify whether someone is at greater risk for developing diseases, how they will respond to treatment, and whether, as well as to what extent, they will recover.