With this new technology, hospitals and pharmacies could make vaccines and medicines onsite
Lina Zeldovich has written about science, medicine and technology for Popular Science, Smithsonian, National Geographic, Scientific American, Reader’s Digest, the New York Times and other major national and international publications. A Columbia J-School alumna, she has won several awards for her stories, including the ASJA Crisis Coverage Award for Covid reporting, and has been a contributing editor at Nautilus Magazine. In 2021, Zeldovich released her first book, The Other Dark Matter, published by the University of Chicago Press, about the science and business of turning waste into wealth and health. You can find her on http://linazeldovich.com/ and @linazeldovich.
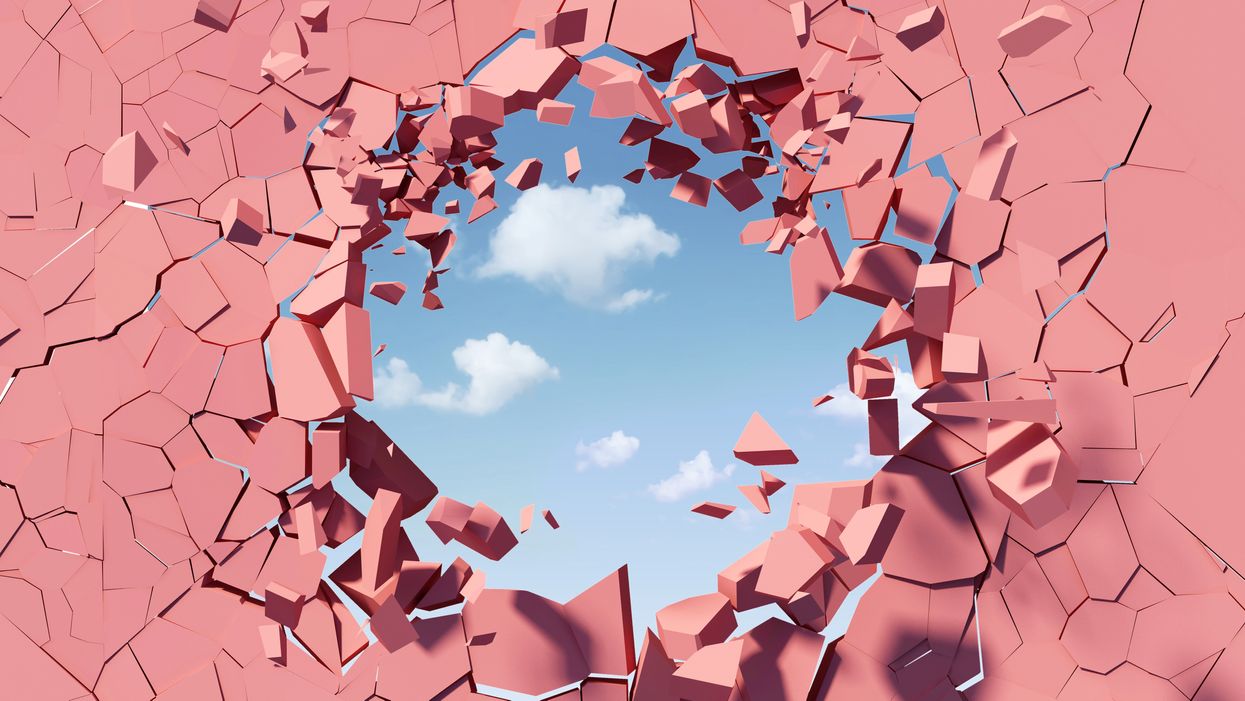
New research focuses on methods that could change medicine-making worldwide. The scientists propose bursting cells open, removing their DNA and using the cellular gears inside to make therapies.
Most modern biopharmaceutical medicines are produced by workhorse cells—typically bacterial but sometimes mammalian. The cells receive the synthesizing instructions on a snippet of a genetic code, which they incorporate into their DNA. The cellular machinery—ribosomes, RNAs, polymerases, and other compounds—read and use these instructions to build the medicinal molecules, which are harvested and administered to patients.
Although a staple of modern pharma, this process is complex and expensive. One must first insert the DNA instructions into the cells, which they may or may not uptake. One then must grow the cells, keeping them alive and well, so that they produce the required therapeutics, which then must be isolated and purified. To make this at scale requires massive bioreactors and big factories from where the drugs are distributed—and may take a while to arrive where they’re needed. “The pandemic showed us that this method is slow and cumbersome,” says Govind Rao, professor of biochemical engineering who directs the Center for Advanced Sensor Technology at the University of Maryland, Baltimore County (UMBC). “We need better methods that can work faster and can work locally where an outbreak is happening.”
Rao and his team of collaborators, which spans multiple research institutions, believe they have a better approach that may change medicine-making worldwide. They suggest forgoing the concept of using living cells as medicine-producers. Instead, they propose breaking the cells and using the remaining cellular gears for assembling the therapeutic compounds. Instead of inserting the DNA into living cells, the team burst them open, and removed their DNA altogether. Yet, the residual molecular machinery of ribosomes, polymerases and other cogwheels still functioned the way it would in a cell. “Now if you drop your DNA drug-making instructions into that soup, this machinery starts making what you need,” Rao explains. “And because you're no longer worrying about living cells, it becomes much simpler and more efficient.” The collaborators detail their cell-free protein synthesis or CFPS method in their recent paper published in preprint BioAxiv.
While CFPS does not use living cells, it still needs the basic building blocks to assemble proteins from—such as amino acids, nucleotides and certain types of enzymes. These are regularly added into this “soup” to keep the molecular factory chugging. “We just mix everything in as a batch and we let it integrate,” says James Robert Swartz, professor of chemical engineering and bioengineering at Stanford University and co-author of the paper. “And we make sure that we provide enough oxygen.” Rao likens the process to making milk from milk powder.
For a variety of reasons—from the field’s general inertia to regulatory approval hurdles—the method hasn’t become mainstream. The pandemic rekindled interest in medicines that can be made quickly and easily, so it drew more attention to the technology.
The idea of a cell-free protein synthesis is older than one might think. Swartz first experimented with it around 1997, when he was a chemical engineer at Genentech. While working on engineering bacteria to make pharmaceuticals, he discovered that there was a limit to what E. coli cells, the workhorse darling of pharma, could do. For example, it couldn’t grow and properly fold some complex proteins. “We tried many genetic engineering approaches, many fermentation, development, and environmental control approaches,” Swartz recalls—to no avail.
“The organism had its own agenda,” he quips. “And because everything was happening within the organism, we just couldn't really change those conditions very easily. Some of them we couldn’t change at all—we didn’t have control.”
It was out of frustration with the defiant bacteria that a new idea took hold. Could the cells be opened instead, so that the protein-forming reactions could be influenced more easily? “Obviously, we’d lose the ability for them to reproduce,” Swartz says. But that also meant that they no longer needed to keep the cells alive and could focus on making the specific reactions happen. “We could take the catalysts, the enzymes, and the more complex catalysts and activate them, make them work together, much as they would in a living cell, but the way we wanted.”
In 1998, Swartz joined Stanford, and began perfecting the biochemistry of the cell-free method, identifying the reactions he wanted to foster and stopping those he didn’t want. He managed to make the idea work, but for a variety of reasons—from the field’s general inertia to regulatory approval hurdles—the method hasn’t become mainstream. The pandemic rekindled interest in medicines that can be made quickly and easily, so it drew more attention to the technology. For their BioArxiv paper, the team tested the method by growing a specific antiviral protein called griffithsin.
First identified by Barry O’Keefe at National Cancer Institute over a decade ago, griffithsin is an antiviral known to interfere with many viruses’ ability to enter cells—including HIV, SARS, SARS-CoV-2, MERS and others. Originally isolated from the red algae Griffithsia, it works differently from antibodies and antibody cocktails.
Most antiviral medicines tend to target the specific receptors that viruses use to gain entry to the cells they infect. For example, SARS-CoV-2 uses the infamous spike protein to latch onto the ACE2 receptor of mammalian cells. The antibodies or other antiviral molecules stick to the spike protein, shutting off its ability to cling onto the ACE2 receptors. Unfortunately, the spike proteins mutate very often, so the medicines lose their potency. On the contrary, griffithsin has the ability to cling to the different parts of viral shells called capsids—namely to the molecules of mannose, a type of sugar. That extra stuff, glued all around the capsid like dead weight, makes it impossible for the virus to squeeze into the cell.
“Every time we have a vaccine or an antibody against a specific SARS-CoV-2 strain, that strain then mutates and so you lose efficacy,” Rao explains. “But griffithsin molecules glom onto the viral capsid, so the capsid essentially becomes a sticky mess and can’t enter the cell.” Mannose molecules also don’t mutate as easily as viruses’ receptors, so griffithsin-based antivirals do not have to be constantly updated. And because mannose molecules are found on many viruses’ capsids, it makes griffithsin “a universal neutralizer,” Rao explains.
“When griffithsin was discovered, we recognized that it held a lot of promise as a potential antiviral agent,” O’Keefe says. In 2010, he published a paper about griffithsin efficacy in neutralizing viruses of the corona family—after the first SARS outbreak in the early 2000s, the scientific community was interested in such antivirals. Yet, griffithsin is still not available as an off-the-shelf product. So during the Covid pandemic, the team experimented with synthesizing griffithsin using the cell-free production method. They were able to generate potent griffithsin in less than 24 hours without having to grow living cells.
The antiviral protein isn't the only type of medicine that can be made cell-free. The proteins needed for vaccine production could also be made the same way. “Such portable, on-demand drug manufacturing platforms can produce antiviral proteins within hours, making them ideal for combating future pandemics,” Rao says. “We would be able to stop the pandemic before it spreads.”
Top: Describes the process used in the study. Bottom: Describes how the new medicines and vaccines could be made at the site of a future viral outbreak.
Image courtesy of Rao and team, sourced from An approach to rapid distributed manufacturing of broad spectrumanti-viral griffithsin using cell-free systems to mitigate pandemics.
Rao’s idea is to perfect the technology to the point that any hospital or pharmacy can load up the media containing molecular factories, mix up the required amino acids, nucleotides and enzymes, and harvest the meds within hours. That will allow making medicines onsite and on demand. “That would be a self-contained production unit, so that you could just ship the production wherever the pandemic is breaking out,” says Swartz.
These units and the meds they produce, will, of course, have to undergo rigorous testing. “The biggest hurdles will be validating these against conventional technology,” Rao says. The biotech industry is risk-averse and prefers the familiar methods. But if this approach works, it may go beyond emergency situations and revolutionize the medicine-making paradigm even outside hospitals and pharmacies. Rao hopes that someday the method might become so mainstream that people may be able to buy and operate such reactors at home. “You can imagine a diabetic patient making insulin that way, or some other drugs,” Rao says. It would work not unlike making baby formula from the mere white powder. Just add water—and some oxygen, too.
Lina Zeldovich has written about science, medicine and technology for Popular Science, Smithsonian, National Geographic, Scientific American, Reader’s Digest, the New York Times and other major national and international publications. A Columbia J-School alumna, she has won several awards for her stories, including the ASJA Crisis Coverage Award for Covid reporting, and has been a contributing editor at Nautilus Magazine. In 2021, Zeldovich released her first book, The Other Dark Matter, published by the University of Chicago Press, about the science and business of turning waste into wealth and health. You can find her on http://linazeldovich.com/ and @linazeldovich.
Often called the window to the soul, the eyes are more sacred than other body parts, at least for some.
Awash in a fluid finely calibrated to keep it alive, a human eye rests inside a transparent cubic device. This ECaBox, or Eyes in a Care Box, is a one-of-a-kind system built by scientists at Barcelona’s Centre for Genomic Regulation (CRG). Their goal is to preserve human eyes for transplantation and related research.
In recent years, scientists have learned to transplant delicate organs such as the liver, lungs or pancreas, but eyes are another story. Even when preserved at the average transplant temperature of 4 Centigrade, they last for 48 hours max. That's one explanation for why transplanting the whole eye isn’t possible—only the cornea, the dome-shaped, outer layer of the eye, can withstand the procedure. The retina, the layer at the back of the eyeball that turns light into electrical signals, which the brain converts into images, is extremely difficult to transplant because it's packed with nerve tissue and blood vessels.
These challenges also make it tough to research transplantation. “This greatly limits their use for experiments, particularly when it comes to the effectiveness of new drugs and treatments,” said Maria Pia Cosma, a biologist at Barcelona’s Centre for Genomic Regulation (CRG), whose team is working on the ECaBox.
Eye transplants are desperately needed, but they're nowhere in sight. About 12.7 million people worldwide need a corneal transplant, which means that only one in 70 people who require them, get them. The gaps are international. Eye banks in the United Kingdom are around 20 percent below the level needed to supply hospitals, while Indian eye banks, which need at least 250,000 corneas per year, collect only around 45 to 50 thousand donor corneas (and of those 60 to 70 percent are successfully transplanted).
As for retinas, it's impossible currently to put one into the eye of another person. Artificial devices can be implanted to restore the sight of patients suffering from severe retinal diseases, but the number of people around the world with such “bionic eyes” is less than 600, while in America alone 11 million people have some type of retinal disease leading to severe vision loss. Add to this an increasingly aging population, commonly facing various vision impairments, and you have a recipe for heavy burdens on individuals, the economy and society. In the U.S. alone, the total annual economic impact of vision problems was $51.4 billion in 2017.
Even if you try growing tissues in the petri dish route into organoids mimicking the function of the human eye, you will not get the physiological complexity of the structure and metabolism of the real thing, according to Cosma. She is a member of a scientific consortium that includes researchers from major institutions from Spain, the U.K., Portugal, Italy and Israel. The consortium has received about $3.8 million from the European Union to pursue innovative eye research. Her team’s goal is to give hope to at least 2.2 billion people across the world afflicted with a vision impairment and 33 million who go through life with avoidable blindness.
Their method? Resuscitating cadaveric eyes for at least a month.
If we succeed, it will be the first intact human model of the eye capable of exploring and analyzing regenerative processes ex vivo. -- Maria Pia Cosma.
“We proposed to resuscitate eyes, that is to restore the global physiology and function of human explanted tissues,” Cosma said, referring to living tissues extracted from the eye and placed in a medium for culture. Their ECaBox is an ex vivo biological system, in which eyes taken from dead donors are placed in an artificial environment, designed to preserve the eye’s temperature and pH levels, deter blood clots, and remove the metabolic waste and toxins that would otherwise spell their demise.
Scientists work on resuscitating eyes in the lab of Maria Pia Cosma.
Courtesy of Maria Pia Cosma.
“One of the great challenges is the passage of the blood in the capillary branches of the eye, what we call long-term perfusion,” Cosma said. Capillaries are an intricate network of very thin blood vessels that transport blood, nutrients and oxygen to cells in the body’s organs and systems. To maintain the garland-shaped structure of this network, sufficient amounts of oxygen and nutrients must be provided through the eye circulation and microcirculation. “Our ambition is to combine perfusion of the vessels with artificial blood," along with using a synthetic form of vitreous, or the gel-like fluid that lets in light and supports the the eye's round shape, Cosma said.
The scientists use this novel setup with the eye submersed in its medium to keep the organ viable, so they can test retinal function. “If we succeed, we will ensure full functionality of a human organ ex vivo. It will be the first intact human model of the eye capable of exploring and analyzing regenerative processes ex vivo,” Cosma added.
A rapidly developing field of regenerative medicine aims to stimulate the body's natural healing processes and restore or replace damaged tissues and organs. But for people with retinal diseases, regenerative medicine progress has been painfully slow. “Experiments on rodents show progress, but the risks for humans are unacceptable,” Cosma said.
The ECaBox could boost progress with regenerative medicine for people with retinal diseases, which has been painfully slow because human experiments involving their eyes are too risky. “We will test emerging treatments while reducing animal research, and greatly accelerate the discovery and preclinical research phase of new possible treatments for vision loss at significantly reduced costs,” Cosma explained. Much less time and money would be wasted during the drug discovery process. Their work may even make it possible to transplant the entire eyeball for those who need it.
“It is a very exciting project,” said Sanjay Sharma, a professor of ophthalmology and epidemiology at Queen's University, in Kingston, Canada. “The ability to explore and monitor regenerative interventions will increasingly be of importance as we develop therapies that can regenerate ocular tissues, including the retina.”
Seemingly, there's no sacred religious text or a holy book prohibiting the practice of eye donation.
But is the world ready for eye transplants? “People are a bit weird or very emotional about donating their eyes as compared to other organs,” Cosma said. And much can be said about the problem of eye donor shortage. Concerns include disfigurement and healthcare professionals’ fear that the conversation about eye donation will upset the departed person’s relatives because of cultural or religious considerations. As just one example, Sharma noted the paucity of eye donations in his home country, Canada.
Yet, experts like Sharma stress the importance of these donations for both the recipients and their family members. “It allows them some psychological benefit in a very difficult time,” he said. So why are global eye banks suffering? Is it because the eyes are the windows to the soul?
Seemingly, there's no sacred religious text or a holy book prohibiting the practice of eye donation. In fact, most major religions of the world permit and support organ transplantation and donation, and by extension eye donation, because they unequivocally see it as an “act of neighborly love and charity.” In Hinduism, the concept of eye donation aligns with the Hindu principle of daan or selfless giving, where individuals donate their organs or body after death to benefit others and contribute to society. In Islam, eye donation is a form of sadaqah jariyah, a perpetual charity, as it can continue to benefit others even after the donor's death.
Meanwhile, Buddhist masters teach that donating an organ gives another person the chance to live longer and practice dharma, the universal law and order, more meaningfully; they also dismiss misunderstandings of the type “if you donate an eye, you’ll be born without an eye in the next birth.” And Christian teachings emphasize the values of love, compassion, and selflessness, all compatible with organ donation, eye donation notwithstanding; besides, those that will have a house in heaven, will get a whole new body without imperfections and limitations.
The explanation for people’s resistance may lie in what Deepak Sarma, a professor of Indian religions and philosophy at Case Western Reserve University in Cleveland, calls “street interpretation” of religious or spiritual dogmas. Consider the mechanism of karma, which is about the causal relation between previous and current actions. “Maybe some Hindus believe there is karma in the eyes and, if the eye gets transplanted into another person, they will have to have that karmic card from now on,” Sarma said. “Even if there is peculiar karma due to an untimely death–which might be interpreted by some as bad karma–then you have the karma of the recipient, which is tremendously good karma, because they have access to these body parts, a tremendous gift,” Sarma said. The overall accumulation is that of good karma: “It’s a beautiful kind of balance,” Sarma said.
For the Jews, Christians, and Muslims who believe in the physical resurrection of the body that will be made new in an afterlife, the already existing body is sacred since it will be the basis of a new refashioned body in an afterlife.---Omar Sultan Haque.
With that said, Sarma believes it is a fallacy to personify or anthropomorphize the eye, which doesn’t have a soul, and stresses that the karma attaches itself to the soul and not the body parts. But for scholars like Omar Sultan Haque—a psychiatrist and social scientist at Harvard Medical School, investigating questions across global health, anthropology, social psychology, and bioethics—the hierarchy of sacredness of body parts is entrenched in human psychology. You cannot equate the pinky toe with the face, he explained.
“The eyes are the window to the soul,” Haque said. “People have a hierarchy of body parts that are considered more sacred or essential to the self or soul, such as the eyes, face, and brain.” In his view, the techno-utopian transhumanist communities (especially those in Silicon Valley) have reduced the totality of a person to a mere material object, a “wet robot” that knows no sacredness or hierarchy of human body parts. “But for the Jews, Christians, and Muslims who believe in the physical resurrection of the body that will be made new in an afterlife, the [already existing] body is sacred since it will be the basis of a new refashioned body in an afterlife,” Haque said. “You cannot treat the body like any old material artifact, or old chair or ragged cloth, just because materialistic, secular ideologies want so,” he continued.
For Cosma and her peers, however, the very definition of what is alive or not is a bit semantic. “As soon as we die, the electrophysiological activity in the eye stops,” she said. “The goal of the project is to restore this activity as soon as possible before the highly complex tissue of the eye starts degrading.” Cosma’s group doesn’t yet know when they will be able to keep the eyes alive and well in the ECaBox, but the consensus is that the sooner the better. Hopefully, the taboos and fears around the eye donations will dissipate around the same time.
As Our AI Systems Get Better, So Must We
In order to build the future we want, we must also become ever better humans, explains the futurist Jamie Metzl in this opinion essay.
As the power and capability of our AI systems increase by the day, the essential question we now face is what constitutes peak human. If we stay where we are while the AI systems we are unleashing continually get better, they will meet and then exceed our capabilities in an ever-growing number of domains. But while some technology visionaries like Elon Musk call for us to slow down the development of AI systems to buy time, this approach alone will simply not work in our hyper-competitive world, particularly when the potential benefits of AI are so great and our frameworks for global governance are so weak. In order to build the future we want, we must also become ever better humans.
The list of activities we once saw as uniquely human where AIs have now surpassed us is long and growing. First, AI systems could beat our best chess players, then our best Go players, then our best champions of multi-player poker. They can see patterns far better than we can, generate medical and other hypotheses most human specialists miss, predict and map out new cellular structures, and even generate beautiful, and, yes, creative, art.
A recent paper by Microsoft researchers analyzing the significant leap in capabilities in OpenAI’s latest AI bot, ChatGPT-4, asserted that the algorithm can “solve novel and difficult tasks that span mathematics, coding, vision, medicine, law, psychology and more, without needing any special prompting.” Calling this functionality “strikingly close to human-level performance,” the authors conclude it “could reasonably be viewed as an early (yet still incomplete) version of an artificial general intelligence (AGI) system.”
The concept of AGI has been around for decades. In its common use, the term suggests a time when individual machines can do many different things at a human level, not just one thing like playing Go or analyzing radiological images. Debating when AGI might arrive, a favorite pastime of computer scientists for years, now has become outdated.
We already have AI algorithms and chatbots that can do lots of different things. Based on the generalist definition, in other words, AGI is essentially already here.
Unfettered by the evolved capacity and storage constraints of our brains, AI algorithms can access nearly all of the digitized cultural inheritance of humanity since the dawn of recorded history and have increasing access to growing pools of digitized biological data from across the spectrum of life.
Once we recognize that both AI systems and humans have unique superpowers, the essential question becomes what each of us can do better than the other and what humans and AIs can best do in active collaboration. The future of our species will depend upon our ability to safely, dynamically, and continually figure that out.
With these ever-larger datasets, rapidly increasing computing and memory power, and new and better algorithms, our AI systems will keep getting better faster than most of us can today imagine. These capabilities have the potential to help us radically improve our healthcare, agriculture, and manufacturing, make our economies more productive and our development more sustainable, and do many important things better.
Soon, they will learn how to write their own code. Like human children, in other words, AI systems will grow up. But even that doesn’t mean our human goose is cooked.
Just like dolphins and dogs, these alternate forms of intelligence will be uniquely theirs, not a lesser or greater version of ours. There are lots of things AI systems can't do and will never be able to do because our AI algorithms, for better and for worse, will never be human. Our embodied human intelligence is its own thing.
Our human intelligence is uniquely ours based on the capacities we have developed in our 3.8-billion-year journey from single cell organisms to us. Our brains and bodies represent continuous adaptations on earlier models, which is why our skeletal systems look like those of lizards and our brains like most other mammals with some extra cerebral cortex mixed in. Human intelligence isn’t just some type of disembodied function but the inextricable manifestation of our evolved physical reality. It includes our sensory analytical skills and all of our animal instincts, intuitions, drives, and perceptions. Disembodied machine intelligence is something different than what we have evolved and possess.
Because of this, some linguists including Noam Chomsky have recently argued that AI systems will never be intelligent as long as they are just manipulating symbols and mathematical tokens without any inherent understanding. Nothing could be further from the truth. Anyone interacting with even first-generation AI chatbots quickly realizes that while these systems are far from perfect or omniscient and can sometimes be stupendously oblivious, they are surprisingly smart and versatile and will get more so… forever. We have little idea even how our own minds work, so judging AI systems based on their output is relatively close to how we evaluate ourselves.
Anyone not awed by the potential of these AI systems is missing the point. AI’s newfound capacities demand that we work urgently to establish norms, standards, and regulations at all levels from local to global to manage the very real risks. Pausing our development of AI systems now doesn’t make sense, however, even if it were possible, because we have no sufficient ways of uniformly enacting such a pause, no plan for how we would use the time, and no common framework for addressing global collective challenges like this.
But if all we feel is a passive awe for these new capabilities, we will also be missing the point.
Human evolution, biology, and cultural history are not just some kind of accidental legacy, disability, or parlor trick, but our inherent superpower. Our ancestors outcompeted rivals for billions of years to make us so well suited to the world we inhabit and helped build. Our social organization at scale has made it possible for us to forge civilizations of immense complexity, engineer biology and novel intelligence, and extend our reach to the stars. Our messy, embodied, intuitive, social human intelligence is roughly mimicable by AI systems but, by definition, never fully replicable by them.
Once we recognize that both AI systems and humans have unique superpowers, the essential question becomes what each of us can do better than the other and what humans and AIs can best do in active collaboration. We still don't know. The future of our species will depend upon our ability to safely, dynamically, and continually figure that out.
As we do, we'll learn that many of our ideas and actions are made up of parts, some of which will prove essentially human and some of which can be better achieved by AI systems. Those in every walk of work and life who most successfully identify the optimal contributions of humans, AIs, and the two together, and who build systems and workflows empowering humans to do human things, machines to do machine things, and humans and machines to work together in ways maximizing the respective strengths of each, will be the champions of the 21st century across all fields.
The dawn of the age of machine intelligence is upon us. It’s a quantum leap equivalent to the domestication of plants and animals, industrialization, electrification, and computing. Each of these revolutions forced us to rethink what it means to be human, how we live, and how we organize ourselves. The AI revolution will happen more suddenly than these earlier transformations but will follow the same general trajectory. Now is the time to aggressively prepare for what is fast heading our way, including by active public engagement, governance, and regulation.
AI systems will not replace us, but, like these earlier technology-driven revolutions, they will force us to become different humans as we co-evolve with our technology. We will never reach peak human in our ongoing evolutionary journey, but we’ve got to manage this transition wisely to build the type of future we’d like to inhabit.
Alongside our ascending AIs, we humans still have a lot of climbing to do.