Genetically Sequencing Healthy Babies Yielded Surprising Results
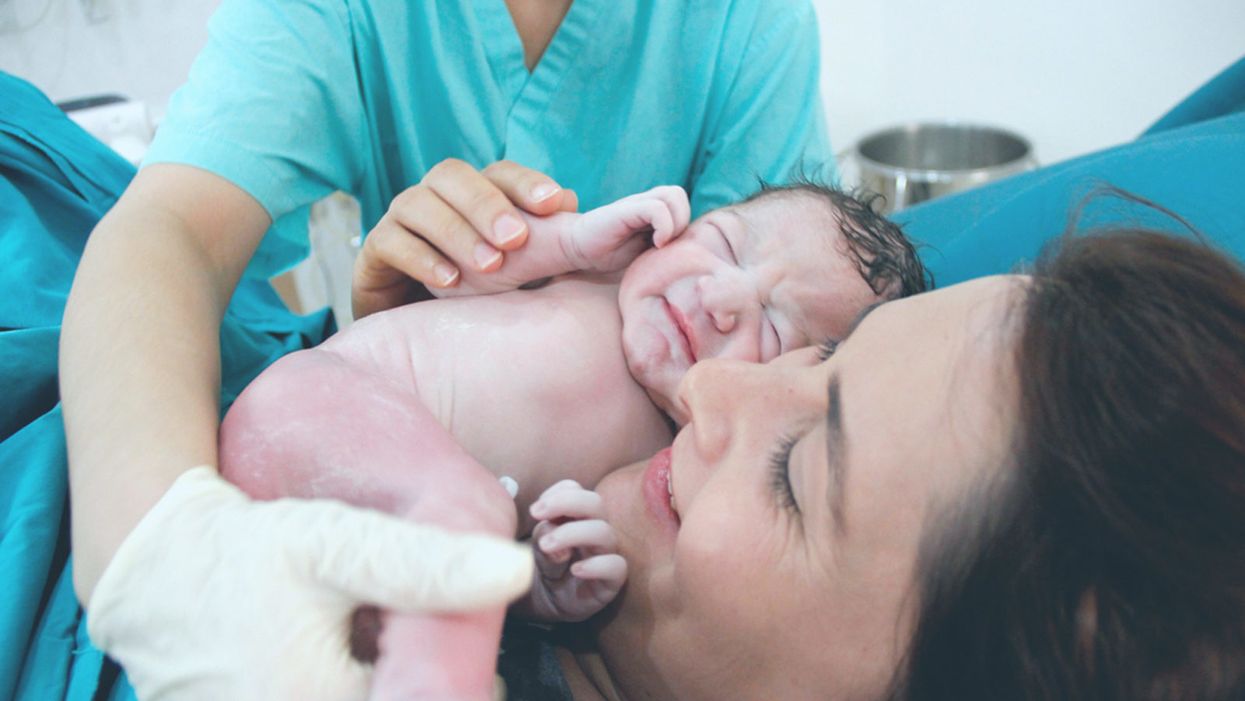
A newborn and mother in the hospital - first touch. (© martin81/Shutterstock)
Today in Melrose, Massachusetts, Cora Stetson is the picture of good health, a bubbly precocious 2-year-old. But Cora has two separate mutations in the gene that produces a critical enzyme called biotinidase and her body produces only 40 percent of the normal levels of that enzyme.
In the last few years, the dream of predicting and preventing diseases through genomics, starting in childhood, is finally within reach.
That's enough to pass conventional newborn (heelstick) screening, but may not be enough for normal brain development, putting baby Cora at risk for seizures and cognitive impairment. But thanks to an experimental study in which Cora's DNA was sequenced after birth, this condition was discovered and she is being treated with a safe and inexpensive vitamin supplement.
Stories like these are beginning to emerge from the BabySeq Project, the first clinical trial in the world to systematically sequence healthy newborn infants. This trial was led by my research group with funding from the National Institutes of Health. While still controversial, it is pointing the way to a future in which adults, or even newborns, can receive comprehensive genetic analysis in order to determine their risk of future disease and enable opportunities to prevent them.
Some believe that medicine is still not ready for genomic population screening, but others feel it is long overdue. After all, the sequencing of the Human Genome Project was completed in 2003, and with this milestone, it became feasible to sequence and interpret the genome of any human being. The costs have come down dramatically since then; an entire human genome can now be sequenced for about $800, although the costs of bioinformatic and medical interpretation can add another $200 to $2000 more, depending upon the number of genes interrogated and the sophistication of the interpretive effort.
Two-year-old Cora Stetson, whose DNA sequencing after birth identified a potentially dangerous genetic mutation in time for her to receive preventive treatment.
(Photo courtesy of Robert Green)
The ability to sequence the human genome yielded extraordinary benefits in scientific discovery, disease diagnosis, and targeted cancer treatment. But the ability of genomes to detect health risks in advance, to actually predict the medical future of an individual, has been mired in controversy and slow to manifest. In particular, the oft-cited vision that healthy infants could be genetically tested at birth in order to predict and prevent the diseases they would encounter, has proven to be far tougher to implement than anyone anticipated.
But in the last few years, the dream of predicting and preventing diseases through genomics, starting in childhood, is finally within reach. Why did it take so long? And what remains to be done?
Great Expectations
Part of the problem was the unrealistic expectations that had been building for years in advance of the genomic science itself. For example, the 1997 film Gattaca portrayed a near future in which the lifetime risk of disease was readily predicted the moment an infant is born. In the fanfare that accompanied the completion of the Human Genome Project, the notion of predicting and preventing future disease in an individual became a powerful meme that was used to inspire investment and public support for genomic research long before the tools were in place to make it happen.
Another part of the problem was the success of state-mandated newborn screening programs that began in the 1960's with biochemical tests of the "heel-stick" for babies with metabolic disorders. These programs have worked beautifully, costing only a few dollars per baby and saving thousands of infants from death and severe cognitive impairment. It seemed only logical that a new technology like genome sequencing would add power and promise to such programs. But instead of embracing the notion of newborn sequencing, newborn screening laboratories have thus far rejected the entire idea as too expensive, too ambiguous, and too threatening to the comfortable constituency that they had built within the public health framework.
"What can you find when you look as deeply as possible into the medical genomes of healthy individuals?"
Creating the Evidence Base for Preventive Genomics
Despite a number of obstacles, there are researchers who are exploring how to achieve the original vision of genomic testing as a tool for disease prediction and prevention. For example, in our NIH-funded MedSeq Project, we were the first to ask the question: "What can you find when you look as deeply as possible into the medical genomes of healthy individuals?"
Most people do not understand that genetic information comes in four separate categories: 1) dominant mutations putting the individual at risk for rare conditions like familial forms of heart disease or cancer, (2) recessive mutations putting the individual's children at risk for rare conditions like cystic fibrosis or PKU, (3) variants across the genome that can be tallied to construct polygenic risk scores for common conditions like heart disease or type 2 diabetes, and (4) variants that can influence drug metabolism or predict drug side effects such as the muscle pain that occasionally occurs with statin use.
The technological and analytical challenges of our study were formidable, because we decided to systematically interrogate over 5000 disease-associated genes and report results in all four categories of genetic information directly to the primary care physicians for each of our volunteers. We enrolled 200 adults and found that everyone who was sequenced had medically relevant polygenic and pharmacogenomic results, over 90 percent carried recessive mutations that could have been important to reproduction, and an extraordinary 14.5 percent carried dominant mutations for rare genetic conditions.
A few years later we launched the BabySeq Project. In this study, we restricted the number of genes to include only those with child/adolescent onset that could benefit medically from early warning, and even so, we found 9.4 percent carried dominant mutations for rare conditions.
At first, our interpretation around the high proportion of apparently healthy individuals with dominant mutations for rare genetic conditions was simple – that these conditions had lower "penetrance" than anticipated; in other words, only a small proportion of those who carried the dominant mutation would get the disease. If this interpretation were to hold, then genetic risk information might be far less useful than we had hoped.
Suddenly the information available in the genome of even an apparently healthy individual is looking more robust, and the prospect of preventive genomics is looking feasible.
But then we circled back with each adult or infant in order to examine and test them for any possible features of the rare disease in question. When we did this, we were surprised to see that in over a quarter of those carrying such mutations, there were already subtle signs of the disease in question that had not even been suspected! Now our interpretation was different. We now believe that genetic risk may be responsible for subclinical disease in a much higher proportion of people than has ever been suspected!
Meanwhile, colleagues of ours have been demonstrating that detailed analysis of polygenic risk scores can identify individuals at high risk for common conditions like heart disease. So adding up the medically relevant results in any given genome, we start to see that you can learn your risks for a rare monogenic condition, a common polygenic condition, a bad effect from a drug you might take in the future, or for having a child with a devastating recessive condition. Suddenly the information available in the genome of even an apparently healthy individual is looking more robust, and the prospect of preventive genomics is looking feasible.
Preventive Genomics Arrives in Clinical Medicine
There is still considerable evidence to gather before we can recommend genomic screening for the entire population. For example, it is important to make sure that families who learn about such risks do not suffer harms or waste resources from excessive medical attention. And many doctors don't yet have guidance on how to use such information with their patients. But our research is convincing many people that preventive genomics is coming and that it will save lives.
In fact, we recently launched a Preventive Genomics Clinic at Brigham and Women's Hospital where information-seeking adults can obtain predictive genomic testing with the highest quality interpretation and medical context, and be coached over time in light of their disease risks toward a healthier outcome. Insurance doesn't yet cover such testing, so patients must pay out of pocket for now, but they can choose from a menu of genetic screening tests, all of which are more comprehensive than consumer-facing products. Genetic counseling is available but optional. So far, this service is for adults only, but sequencing for children will surely follow soon.
As the costs of sequencing and other Omics technologies continue to decline, we will see both responsible and irresponsible marketing of genetic testing, and we will need to guard against unscientific claims. But at the same time, we must be far more imaginative and fast moving in mainstream medicine than we have been to date in order to claim the emerging benefits of preventive genomics where it is now clear that suffering can be averted, and lives can be saved. The future has arrived if we are bold enough to grasp it.
Funding and Disclosures:
Dr. Green's research is supported by the National Institutes of Health, the Department of Defense and through donations to The Franca Sozzani Fund for Preventive Genomics. Dr. Green receives compensation for advising the following companies: AIA, Applied Therapeutics, Helix, Ohana, OptraHealth, Prudential, Verily and Veritas; and is co-founder and advisor to Genome Medical, Inc, a technology and services company providing genetics expertise to patients, providers, employers and care systems.
Waste smothering our oceans is worth billions – here’s what we can do with all that sh$t
In 2015, human poop was valued at $9.5 billion per year, which today would be $11.5 billion. The Ocean Sewage Alliance is uniting experts from key sectors to change how we handle our sewage and, in the process, create all sorts of economic benefits.
There’s hardly a person out there who hasn’t heard of the Great Pacific Garbage Patch. That type of pollution is impossible to miss. It stares you in the face from pictures and videos of sea turtles with drinking straws up their noses and acres of plastic swirling in the sea.
It demands you to solve the problem—and it works. The campaign to raise awareness about plastic pollution in the oceans has resulted in new policies, including bans on microplastics in personal care products, technology to clean up the plastic, and even new plastic-like materials that are better for the environment.
But there’s a different type of pollution smothering the ocean as you read this. Unfortunately, this one is almost invisible, but no less damaging. In fact, it’s even more serious than plastic and most people have no idea it even exists. It is literally under our noses, destroying our oceans, lakes, and rivers – and yet we are missing it completely while contributing to it daily. In fact, we exacerbate it multiple times a day—every time we use the bathroom.
It is the way we do our sewage.
Most of us don’t think much about what happens after we flush the toilet. Most of us probably assume that the substances we flush go “somewhere” and are dealt with safely. But we typically don’t think about it beyond that.
Most of us also probably don’t think about what’s in the ocean or lakes we swim in. Since others are swimming, jumping in is just fine. But our waterways are far from clean. In fact, at times they are incredibly filthy. In the US, we are dumping 1.2 trillion of gallons of untreated sewage into the environment every year. Just New York City alone discharges 27 billion gallons into the Hudson River basin annually.
How does this happen? Part of it is the unfortunate side effect of our sewage system design that dates back to over a century ago when cities were smaller and fewer people were living so close together.
Back then, engineers designed the so-called “combine sewer overflow systems,” or CSOs, in which the storm water pipes are connected to the sanitary sewer pipes. In normal conditions, the sewage effluent from homes flows to the treatment plants where it gets cleaned and released into the waterways. But when it rains, the pipe system becomes so overwhelmed with water that the treatment plant can’t process it fast enough. So the treatment plant has to release the excess water through its discharge pipes—directly, without treatment, into streams, rivers and the ocean.
The 1.2 trillion gallons of CSO releases isn’t even the full picture. There are also discharges from poorly maintained septic systems, cesspools and busted pipes of the aging wastewater infrastructure. The state of Hawaii alone has 88,000 cesspools that need replacing and are currently leaking 53 million gallons of raw sewage daily into their coastal waters. You may think twice about swimming on your Hawaii vacations.
Overall, the US is facing a $271 billion backlog in wastewater infrastructure projects to update these aging systems. Across the Western world, countries are facing similar challenges with their aging sewage systems, especially the UK and European Union.
That’s not to say that other parts of the planet are in better shape. Out of the 7+ billion people populating our earth, 4.2 billion don’t have access to safe sanitation. Included in this insane number are roughly 2 billion people who have no toilet at all. Whether washed by rains or dumped directly into the waterways, a lot of this sludge pollutes the environment, the drinking water, and ultimately the ocean.
Pipes pour water onto a rocky shore in Jakarta, Indonesia.
Tom Fisk
What complicates this from an ocean health perspective is that it’s not just poop and pee that gets dumped into nearby waterways. It is all the things we put in and on our bodies and flush down our drains. That vicious mix of chemicals includes caffeine, antibiotics, antidepressants, painkillers, hormones, microplastics, cocaine, cooking oils, paint thinners, and PFAS—the forever chemicals present in everything from breathable clothing to fire retardant fabrics of our living room couches. Recent reports have found all of the above substances in fish—and then some.
Why do we allow so much untreated sewage spill into the sea? Frankly speaking, for decades scientists and engineers thought that the ocean could handle it. The mantra back then was “dilution is the solution to pollution,” which might’ve worked when there were much fewer people living on earth—but not now. Today science is telling us that this old approach doesn’t hold. That marine habitats are much more sensitive than we had expected and can’t handle the amount of wastewater we are discharging into them.
The excess nitrogen and phosphorus that the sewage (and agricultural runoff) dumps into the water causes harmful algal blooms, more commonly known as red or brown tides. The water column is overtaken by tiny algae that sucks up all the oxygen from the water, creating dead zones like the big fish kills in the Gulf of Mexico. These algae also cause public health issues by releasing gases toxic to people and animals, including dementia, neurological damage, and respiratory illness. Marshes and mangroves end up with weakened root systems and start dying off. In a wastewater modeling study I published last year, we found that 31 percent of salt marshes globally were heavily polluted with human sewage. Coral reefs get riddled with disease and overgrown by seaweed.
We could convert sewage into high-value goods. It can be used to generate electricity, fertilizer, and drinking water. The technologies not only exist but are getting better and more efficient all the time.
Moreover, by way of our sewage, we managed to transmit a human pathogen—Serratia marcescens, which causes urinary, respiratory and other infections in people—to corals! Recent reports from the Florida Keys are showing white pox disease popping up in elk horn corals caused by S.marcescens, which somehow managed to jump species. Many recent studies have documented just how common this type of pollution is across the globe.
Yet, there is some good news in that abysmal sewage flow. Just like with plastic pollution, realizing that there’s a problem is the first step, so awareness is key. That’s exactly why I co-founded Ocean Sewage Alliance last year—a nonprofit that aims to “re-potty train the world” by breaking taboos in talking about the poop and pee problem, as well as uniting experts from various key sectors to work together to end sewage pollution in coastal areas.
To end this pollution, we have to change the ways we handle our sewage. Even more exciting is that by solving the sewage problem we can create all sorts of economic benefits. In 2015, human poop was valued at $9.5 billion a year globally, which today would be $11.5 billion per year.
What would one do with that sh$t?
We could convert it into high-value goods. Sewage can be used to generate electricity, fertilizer, and drinking water. The technologies not only exist but are getting better and more efficient all the time. Some exciting examples include biodigesters and urine diversion (or peecycling) systems that can produce fertilizer and biogas, essentially natural gas. The United Nations estimates that the biogas produced from poop could provide electricity for 138 million homes. And the recovered and cleaned water can be used for irrigation, laundry and flushing toilets. It can even be refined to the point that it is safe for drinking water – just ask the folks in Orange County, CA who have been doing so for the last few decades.
How do we deal with all the human-made pollutants in our sewage? There is technology for that too. Called pyrolysis, it heats up sludge to high temperatures in the absence of oxygen, which causes most of the substances to degrade and fall apart.
There are solutions to the problems—as long as we acknowledge that the problems exist. The fact that you are reading this means that you are part of the solution already. The next time you flush your toilet, think about where this output may flow. Does your septic system work properly? Does your local treatment plant discharge raw sewage on rainy days? Can that plant implement newer technologies that can upcycle waste? These questions are part of re-potty training the world, one household at a time. And together, these households are the force that can turn back the toxic sewage tide. And keep our oceans blue.
The U.S. must fund more biotech innovation – or other countries will catch up faster than you think
In the coming years, U.S. market share in biotech will decline unless the federal government makes investments to improve the quality and quantity of U.S. research, writes the author.
The U.S. has approximately 58 percent of the market share in the biotech sector, followed by China with 11 percent. However, this market share is the result of several years of previous research and development (R&D) – it is a present picture of what happened in the past. In the future, this market share will decline unless the federal government makes investments to improve the quality and quantity of U.S. research in biotech.
The effectiveness of current R&D can be evaluated in a variety of ways such as monies invested and the number of patents filed. According to the UNESCO Institute for Statistics, the U.S. spends approximately 2.7 percent of GDP on R&D ($476,459.0M), whereas China spends 2 percent ($346,266.3M). However, investment levels do not necessarily translate into goods that end up contributing to innovation.
Patents are a better indication of innovation. The biotech industry relies on patents to protect their investments, making patenting a key tool in the process of translating scientific discoveries that can ultimately benefit patients. In 2020, China filed 1,497,159 patents, a 6.9 percent increase in growth rate. In contrast, the U.S. filed 597,172, a 3.9 percent decline. When it comes to patents filed, China has approximately 45 percent of the world share compared to 18 percent for the U.S.
So how did we get here? The nature of science in academia allows scientists to specialize by dedicating several years to advance discovery research and develop new inventions that can then be licensed by biotech companies. This makes academic science critical to innovation in the U.S. and abroad.
Academic scientists rely on government and foundation grants to pay for R&D, which includes salaries for faculty, investigators and trainees, as well as monies for infrastructure, support personnel and research supplies. Of particular interest to academic scientists to cover these costs is government support such as Research Project Grants, also known as R01 grants, the oldest grant mechanism from the National Institutes of Health. Unfortunately, this funding mechanism is extremely competitive, as applications have a success rate of only about 20 percent. To maximize the chances of getting funded, investigators tend to limit the innovation of their applications, since a project that seems overambitious is discouraged by grant reviewers.
Considering the difficulty in obtaining funding, the limited number of opportunities for scientists to become independent investigators capable of leading their own scientific projects, and the salaries available to pay for scientists with a doctoral degree, it is not surprising that the U.S. is progressively losing its workforce for innovation.
This approach affects the future success of the R&D enterprise in the U.S. Pursuing less innovative work tends to produce scientific results that are more obvious than groundbreaking, and when a discovery is obvious, it cannot be patented, resulting in fewer inventions that go on to benefit patients. Even though there are governmental funding options available for scientists in academia focused on more groundbreaking and translational projects, those options are less coveted by academic scientists who are trying to obtain tenure and long-term funding to cover salaries and other associated laboratory expenses. Therefore, since only a small percent of projects gets funded, the likelihood of scientists interested in pursuing academic science or even research in general keeps declining over time.
Efforts to raise the number of individuals who pursue a scientific education are paying off. However, the number of job openings for those trainees to carry out independent scientific research once they graduate has proved harder to increase. These limitations are not just in the number of faculty openings to pursue academic science, which are in part related to grant funding, but also the low salary available to pay those scientists after they obtain their doctoral degree, which ranges from $53,000 to $65,000, depending on years of experience.
Thus, considering the difficulty in obtaining funding, the limited number of opportunities for scientists to become independent investigators capable of leading their own scientific projects, and the salaries available to pay for scientists with a doctoral degree, it is not surprising that the U.S. is progressively losing its workforce for innovation, which results in fewer patents filed.
Perhaps instead of encouraging scientists to propose less innovative projects in order to increase their chances of getting grants, the U.S. government should give serious consideration to funding investigators for their potential for success -- or the success they have already achieved in contributing to the advancement of science. Such a funding approach should be tiered depending on career stage or years of experience, considering that 42 years old is the median age at which the first R01 is obtained. This suggests that after finishing their training, scientists spend 10 years before they establish themselves as independent academic investigators capable of having the appropriate funds to train the next generation of scientists who will help the U.S. maintain or even expand its market share in the biotech industry for years to come. Patenting should be given more weight as part of the academic endeavor for promotion purposes, or governmental investment in research funding should be increased to support more than just 20 percent of projects.
Remaining at the forefront of biotech innovation will give us the opportunity to not just generate more jobs, but it will also allow us to attract the brightest scientists from all over the world. This talented workforce will go on to train future U.S. scientists and will improve our standard of living by giving us the opportunity to produce the next generation of therapies intended to improve human health.
This problem cannot rely on just one solution, but what is certain is that unless there are more creative changes in funding approaches for scientists in academia, eventually we may be saying “remember when the U.S. was at the forefront of biotech innovation?”