Genetically Sequencing Healthy Babies Yielded Surprising Results
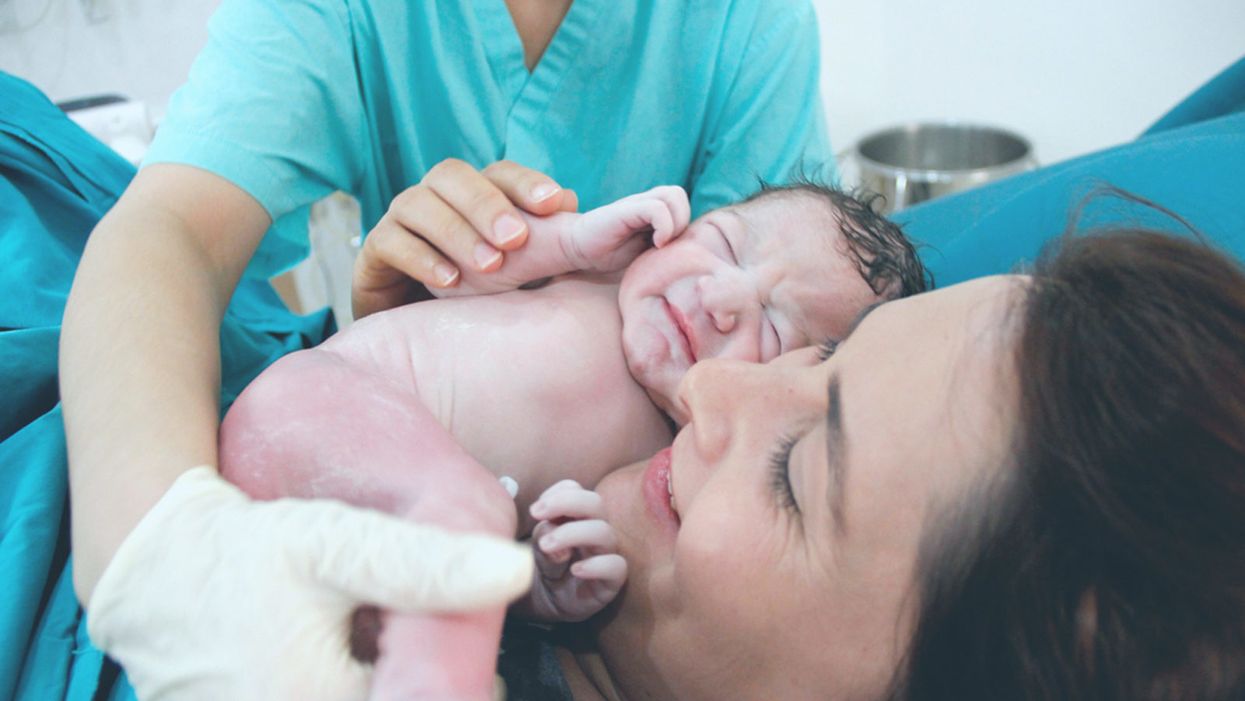
A newborn and mother in the hospital - first touch. (© martin81/Shutterstock)
Today in Melrose, Massachusetts, Cora Stetson is the picture of good health, a bubbly precocious 2-year-old. But Cora has two separate mutations in the gene that produces a critical enzyme called biotinidase and her body produces only 40 percent of the normal levels of that enzyme.
In the last few years, the dream of predicting and preventing diseases through genomics, starting in childhood, is finally within reach.
That's enough to pass conventional newborn (heelstick) screening, but may not be enough for normal brain development, putting baby Cora at risk for seizures and cognitive impairment. But thanks to an experimental study in which Cora's DNA was sequenced after birth, this condition was discovered and she is being treated with a safe and inexpensive vitamin supplement.
Stories like these are beginning to emerge from the BabySeq Project, the first clinical trial in the world to systematically sequence healthy newborn infants. This trial was led by my research group with funding from the National Institutes of Health. While still controversial, it is pointing the way to a future in which adults, or even newborns, can receive comprehensive genetic analysis in order to determine their risk of future disease and enable opportunities to prevent them.
Some believe that medicine is still not ready for genomic population screening, but others feel it is long overdue. After all, the sequencing of the Human Genome Project was completed in 2003, and with this milestone, it became feasible to sequence and interpret the genome of any human being. The costs have come down dramatically since then; an entire human genome can now be sequenced for about $800, although the costs of bioinformatic and medical interpretation can add another $200 to $2000 more, depending upon the number of genes interrogated and the sophistication of the interpretive effort.
Two-year-old Cora Stetson, whose DNA sequencing after birth identified a potentially dangerous genetic mutation in time for her to receive preventive treatment.
(Photo courtesy of Robert Green)
The ability to sequence the human genome yielded extraordinary benefits in scientific discovery, disease diagnosis, and targeted cancer treatment. But the ability of genomes to detect health risks in advance, to actually predict the medical future of an individual, has been mired in controversy and slow to manifest. In particular, the oft-cited vision that healthy infants could be genetically tested at birth in order to predict and prevent the diseases they would encounter, has proven to be far tougher to implement than anyone anticipated.
But in the last few years, the dream of predicting and preventing diseases through genomics, starting in childhood, is finally within reach. Why did it take so long? And what remains to be done?
Great Expectations
Part of the problem was the unrealistic expectations that had been building for years in advance of the genomic science itself. For example, the 1997 film Gattaca portrayed a near future in which the lifetime risk of disease was readily predicted the moment an infant is born. In the fanfare that accompanied the completion of the Human Genome Project, the notion of predicting and preventing future disease in an individual became a powerful meme that was used to inspire investment and public support for genomic research long before the tools were in place to make it happen.
Another part of the problem was the success of state-mandated newborn screening programs that began in the 1960's with biochemical tests of the "heel-stick" for babies with metabolic disorders. These programs have worked beautifully, costing only a few dollars per baby and saving thousands of infants from death and severe cognitive impairment. It seemed only logical that a new technology like genome sequencing would add power and promise to such programs. But instead of embracing the notion of newborn sequencing, newborn screening laboratories have thus far rejected the entire idea as too expensive, too ambiguous, and too threatening to the comfortable constituency that they had built within the public health framework.
"What can you find when you look as deeply as possible into the medical genomes of healthy individuals?"
Creating the Evidence Base for Preventive Genomics
Despite a number of obstacles, there are researchers who are exploring how to achieve the original vision of genomic testing as a tool for disease prediction and prevention. For example, in our NIH-funded MedSeq Project, we were the first to ask the question: "What can you find when you look as deeply as possible into the medical genomes of healthy individuals?"
Most people do not understand that genetic information comes in four separate categories: 1) dominant mutations putting the individual at risk for rare conditions like familial forms of heart disease or cancer, (2) recessive mutations putting the individual's children at risk for rare conditions like cystic fibrosis or PKU, (3) variants across the genome that can be tallied to construct polygenic risk scores for common conditions like heart disease or type 2 diabetes, and (4) variants that can influence drug metabolism or predict drug side effects such as the muscle pain that occasionally occurs with statin use.
The technological and analytical challenges of our study were formidable, because we decided to systematically interrogate over 5000 disease-associated genes and report results in all four categories of genetic information directly to the primary care physicians for each of our volunteers. We enrolled 200 adults and found that everyone who was sequenced had medically relevant polygenic and pharmacogenomic results, over 90 percent carried recessive mutations that could have been important to reproduction, and an extraordinary 14.5 percent carried dominant mutations for rare genetic conditions.
A few years later we launched the BabySeq Project. In this study, we restricted the number of genes to include only those with child/adolescent onset that could benefit medically from early warning, and even so, we found 9.4 percent carried dominant mutations for rare conditions.
At first, our interpretation around the high proportion of apparently healthy individuals with dominant mutations for rare genetic conditions was simple – that these conditions had lower "penetrance" than anticipated; in other words, only a small proportion of those who carried the dominant mutation would get the disease. If this interpretation were to hold, then genetic risk information might be far less useful than we had hoped.
Suddenly the information available in the genome of even an apparently healthy individual is looking more robust, and the prospect of preventive genomics is looking feasible.
But then we circled back with each adult or infant in order to examine and test them for any possible features of the rare disease in question. When we did this, we were surprised to see that in over a quarter of those carrying such mutations, there were already subtle signs of the disease in question that had not even been suspected! Now our interpretation was different. We now believe that genetic risk may be responsible for subclinical disease in a much higher proportion of people than has ever been suspected!
Meanwhile, colleagues of ours have been demonstrating that detailed analysis of polygenic risk scores can identify individuals at high risk for common conditions like heart disease. So adding up the medically relevant results in any given genome, we start to see that you can learn your risks for a rare monogenic condition, a common polygenic condition, a bad effect from a drug you might take in the future, or for having a child with a devastating recessive condition. Suddenly the information available in the genome of even an apparently healthy individual is looking more robust, and the prospect of preventive genomics is looking feasible.
Preventive Genomics Arrives in Clinical Medicine
There is still considerable evidence to gather before we can recommend genomic screening for the entire population. For example, it is important to make sure that families who learn about such risks do not suffer harms or waste resources from excessive medical attention. And many doctors don't yet have guidance on how to use such information with their patients. But our research is convincing many people that preventive genomics is coming and that it will save lives.
In fact, we recently launched a Preventive Genomics Clinic at Brigham and Women's Hospital where information-seeking adults can obtain predictive genomic testing with the highest quality interpretation and medical context, and be coached over time in light of their disease risks toward a healthier outcome. Insurance doesn't yet cover such testing, so patients must pay out of pocket for now, but they can choose from a menu of genetic screening tests, all of which are more comprehensive than consumer-facing products. Genetic counseling is available but optional. So far, this service is for adults only, but sequencing for children will surely follow soon.
As the costs of sequencing and other Omics technologies continue to decline, we will see both responsible and irresponsible marketing of genetic testing, and we will need to guard against unscientific claims. But at the same time, we must be far more imaginative and fast moving in mainstream medicine than we have been to date in order to claim the emerging benefits of preventive genomics where it is now clear that suffering can be averted, and lives can be saved. The future has arrived if we are bold enough to grasp it.
Funding and Disclosures:
Dr. Green's research is supported by the National Institutes of Health, the Department of Defense and through donations to The Franca Sozzani Fund for Preventive Genomics. Dr. Green receives compensation for advising the following companies: AIA, Applied Therapeutics, Helix, Ohana, OptraHealth, Prudential, Verily and Veritas; and is co-founder and advisor to Genome Medical, Inc, a technology and services company providing genetics expertise to patients, providers, employers and care systems.
Regenerative medicine has come a long way, baby
After a cloned baby sheep, what started as one of the most controversial areas in medicine is now promising to transform it.
The field of regenerative medicine had a shaky start. In 2002, when news spread about the first cloned animal, Dolly the sheep, a raucous debate ensued. Scary headlines and organized opposition groups put pressure on government leaders, who responded by tightening restrictions on this type of research.
Fast forward to today, and regenerative medicine, which focuses on making unhealthy tissues and organs healthy again, is rewriting the code to healing many disorders, though it’s still young enough to be considered nascent. What started as one of the most controversial areas in medicine is now promising to transform it.
Progress in the lab has addressed previous concerns. Back in the early 2000s, some of the most fervent controversy centered around somatic cell nuclear transfer (SCNT), the process used by scientists to produce Dolly. There was fear that this technique could be used in humans, with possibly adverse effects, considering the many medical problems of the animals who had been cloned.
But today, scientists have discovered better approaches with fewer risks. Pioneers in the field are embracing new possibilities for cellular reprogramming, 3D organ printing, AI collaboration, and even growing organs in space. It could bring a new era of personalized medicine for longer, healthier lives - while potentially sparking new controversies.
Engineering tissues from amniotic fluids
Work in regenerative medicine seeks to reverse damage to organs and tissues by culling, modifying and replacing cells in the human body. Scientists in this field reach deep into the mechanisms of diseases and the breakdowns of cells, the little workhorses that perform all life-giving processes. If cells can’t do their jobs, they take whole organs and systems down with them. Regenerative medicine seeks to harness the power of healthy cells derived from stem cells to do the work that can literally restore patients to a state of health—by giving them healthy, functioning tissues and organs.
Modern-day regenerative medicine takes its origin from the 1998 isolation of human embryonic stem cells, first achieved by John Gearhart at Johns Hopkins University. Gearhart isolated the pluripotent cells that can differentiate into virtually every kind of cell in the human body. There was a raging controversy about the use of these cells in research because at that time they came exclusively from early-stage embryos or fetal tissue.
Back then, the highly controversial SCNT cells were the only way to produce genetically matched stem cells to treat patients. Since then, the picture has changed radically because other sources of highly versatile stem cells have been developed. Today, scientists can derive stem cells from amniotic fluid or reprogram patients’ skin cells back to an immature state, so they can differentiate into whatever types of cells the patient needs.
In the context of medical history, the field of regenerative medicine is progressing at a dizzying speed. But for those living with aggressive or chronic illnesses, it can seem that the wheels of medical progress grind slowly.
The ethical debate has been dialed back and, in the last few decades, the field has produced important innovations, spurring the development of whole new FDA processes and categories, says Anthony Atala, a bioengineer and director of the Wake Forest Institute for Regenerative Medicine. Atala and a large team of researchers have pioneered many of the first applications of 3D printed tissues and organs using cells developed from patients or those obtained from amniotic fluid or placentas.
His lab, considered to be the largest devoted to translational regenerative medicine, is currently working with 40 different engineered human tissues. Sixteen of them have been transplanted into patients. That includes skin, bladders, urethras, muscles, kidneys and vaginal organs, to name just a few.
These achievements are made possible by converging disciplines and technologies, such as cell therapies, bioengineering, gene editing, nanotechnology and 3D printing, to create living tissues and organs for human transplants. Atala is currently overseeing clinical trials to test the safety of tissues and organs engineered in the Wake Forest lab, a significant step toward FDA approval.
In the context of medical history, the field of regenerative medicine is progressing at a dizzying speed. But for those living with aggressive or chronic illnesses, it can seem that the wheels of medical progress grind slowly.
“It’s never fast enough,” Atala says. “We want to get new treatments into the clinic faster, but the reality is that you have to dot all your i’s and cross all your t’s—and rightly so, for the sake of patient safety. People want predictions, but you can never predict how much work it will take to go from conceptualization to utilization.”
As a surgeon, he also treats patients and is able to follow transplant recipients. “At the end of the day, the goal is to get these technologies into patients, and working with the patients is a very rewarding experience,” he says. Will the 3D printed organs ever outrun the shortage of donated organs? “That’s the hope,” Atala says, “but this technology won’t eliminate the need for them in our lifetime.”
New methods are out of this world
Jeanne Loring, another pioneer in the field and director of the Center for Regenerative Medicine at Scripps Research Institute in San Diego, says that investment in regenerative medicine is not only paying off, but is leading to truly personalized medicine, one of the holy grails of modern science.
This is because a patient’s own skin cells can be reprogrammed to become replacements for various malfunctioning cells causing incurable diseases, such as diabetes, heart disease, macular degeneration and Parkinson’s. If the cells are obtained from a source other than the patient, they can be rejected by the immune system. This means that patients need lifelong immunosuppression, which isn’t ideal. “With Covid,” says Loring, “I became acutely aware of the dangers of immunosuppression.” Using the patient’s own cells eliminates that problem.
Microgravity conditions make it easier for the cells to form three-dimensional structures, which could more easily lead to the growing of whole organs. In fact, Loring's own cells have been sent to the ISS for study.
Loring has a special interest in neurons, or brain cells that can be developed by manipulating cells found in the skin. She is looking to eventually treat Parkinson’s disease using them. The manipulated cells produce dopamine, the critical hormone or neurotransmitter lacking in the brains of patients. A company she founded plans to start a Phase I clinical trial using cell therapies for Parkinson’s soon, she says.
This is the culmination of many years of basic research on her part, some of it on her own cells. In 2007, Loring had her own cells reprogrammed, so there’s a cell line that carries her DNA. “They’re just like embryonic stem cells, but personal,” she said.
Loring has another special interest—sending immature cells into space to be studied at the International Space Station. There, microgravity conditions make it easier for the cells to form three-dimensional structures, which could more easily lead to the growing of whole organs. In fact, her own cells have been sent to the ISS for study. “My colleagues and I have completed four missions at the space station,” she says. “The last cells came down last August. They were my own cells reprogrammed into pluripotent cells in 2009. No one else can say that,” she adds.
Future controversies and tipping points
Although the original SCNT debate has calmed down, more controversies may arise, Loring thinks.
One of them could concern growing synthetic embryos. The embryos are ultimately derived from embryonic stem cells, and it’s not clear to what stage these embryos can or will be grown in an artificial uterus—another recent invention. The science, so far done only in animals, is still new and has not been widely publicized but, eventually, “People will notice the production of synthetic embryos and growing them in an artificial uterus,” Loring says. It’s likely to incite many of the same reactions as the use of embryonic stem cells.
Bernard Siegel, the founder and director of the Regenerative Medicine Foundation and executive director of the newly formed Healthspan Action Coalition (HSAC), believes that stem cell science is rapidly approaching tipping point and changing all of medical science. (For disclosure, I do consulting work for HSAC). Siegel says that regenerative medicine has become a new pillar of medicine that has recently been fast-tracked by new technology.
Artificial intelligence is speeding up discoveries and the convergence of key disciplines, as demonstrated in Atala’s lab, which is creating complex new medical products that replace the body’s natural parts. Just as importantly, those parts are genetically matched and pose no risk of rejection.
These new technologies must be regulated, which can be a challenge, Siegel notes. “Cell therapies represent a challenge to the existing regulatory structure, including payment, reimbursement and infrastructure issues that 20 years ago, didn’t exist.” Now the FDA and other agencies are faced with this revolution, and they’re just beginning to adapt.
Siegel cited the 2021 FDA Modernization Act as a major step. The Act allows drug developers to use alternatives to animal testing in investigating the safety and efficacy of new compounds, loosening the agency’s requirement for extensive animal testing before a new drug can move into clinical trials. The Act is a recognition of the profound effect that cultured human cells are having on research. Being able to test drugs using actual human cells promises to be far safer and more accurate in predicting how they will act in the human body, and could accelerate drug development.
Siegel, a longtime veteran and founding father of several health advocacy organizations, believes this work helped bring cell therapies to people sooner rather than later. His new focus, through the HSAC, is to leverage regenerative medicine into extending not just the lifespan but the worldwide human healthspan, the period of life lived with health and vigor. “When you look at the HSAC as a tree,” asks Siegel, “what are the roots of that tree? Stem cell science and the huge ecosystem it has created.” The study of human aging is another root to the tree that has potential to lengthen healthspans.
The revolutionary science underlying the extension of the healthspan needs to be available to the whole world, Siegel says. “We need to take all these roots and come up with a way to improve the life of all mankind,” he says. “Everyone should be able to take advantage of this promising new world.”
Joy Milne's unusual sense of smell led Dr. Tilo Kunath, a neurobiologist at the Centre for Regenerative Medicine at the University of Edinburgh, and a host of other scientists, to develop a new diagnostic test for Parkinson's.
Forty years ago, Joy Milne, a nurse from Perth, Scotland, noticed a musky odor coming from her husband, Les. At first, Milne thought the smell was a result of bad hygiene and badgered her husband to take longer showers. But when the smell persisted, Milne learned to live with it, not wanting to hurt her husband's feelings.
Twelve years after she first noticed the "woodsy" smell, Les was diagnosed at the age of 44 with Parkinson's Disease, a neurodegenerative condition characterized by lack of dopamine production and loss of movement. Parkinson's Disease currently affects more than 10 million people worldwide.
Milne spent the next several years believing the strange smell was exclusive to her husband. But to her surprise, at a local support group meeting in 2012, she caught the familiar scent once again, hanging over the group like a cloud. Stunned, Milne started to wonder if the smell was the result of Parkinson's Disease itself.
Milne's discovery led her to Dr. Tilo Kunath, a neurobiologist at the Centre for Regenerative Medicine at the University of Edinburgh. Together, Milne, Kunath, and a host of other scientists would use Milne's unusual sense of smell to develop a new diagnostic test, now in development and poised to revolutionize the treatment of Parkinson's Disease.
"Joy was in the audience during a talk I was giving on my work, which has to do with Parkinson's and stem cell biology," Kunath says. "During the patient engagement portion of the talk, she asked me if Parkinson's had a smell to it." Confused, Kunath said he had never heard of this – but for months after his talk he continued to turn the question over in his mind.
Kunath knew from his research that the skin's microbiome changes during different disease processes, releasing metabolites that can give off odors. In the medical literature, diseases like melanoma and Type 2 diabetes have been known to carry a specific scent – but no such connection had been made with Parkinson's. If people could smell Parkinson's, he thought, then it stood to reason that those metabolites could be isolated, identified, and used to potentially diagnose Parkinson's by their presence alone.
First, Kunath and his colleagues decided to test Milne's sense of smell. "I got in touch with Joy again and we designed a protocol to test her sense of smell without her having to be around patients," says Kunath, which could have affected the validity of the test. In his spare time, Kunath collected t-shirt samples from people diagnosed with Parkinson's and from others without the diagnosis and gave them to Milne to smell. In 100 percent of the samples, Milne was able to detect whether a person had Parkinson's based on smell alone. Amazingly, Milne was even able to detect the "Parkinson's scent" in a shirt from the control group – someone who did not have a Parkinson's diagnosis, but would go on to be diagnosed nine months later.
From the initial study, the team discovered that Parkinson's did have a smell, that Milne – inexplicably – could detect it, and that she could detect it long before diagnosis like she had with her husband, Les. But the experiments revealed other things that the team hadn't been expecting.
"One surprising thing we learned from that experiment was that the odor was always located in the back of the shirt – never in the armpit, where we expected the smell to be," Kunath says. "I had a chance meeting with a dermatologist and he said the smell was due to the patient's sebum, which are greasy secretions that are really dense on your upper back. We have sweat glands, instead of sebum, in our armpits." Patients with Parkinson's are also known to have increased sebum production.
With the knowledge that a patient's sebum was the source of the unusual smell, researchers could go on to investigate exactly what metabolites were in the sebum and in what amounts. Kunath, along with his associate, Dr. Perdita Barran, collected and analyzed sebum samples from 64 participants across the United Kingdom. Once the samples were collected, Barran and others analyzed it using a method called gas chromatography mass spectrometry, or GS-MC, which separated, weighed and helped identify the individual compounds present in each sebum sample.
Barran's team can now correctly identify Parkinson's in nine out of 10 patients – a much quicker and more accurate way to diagnose than what clinicians do now.
"The compounds we've identified in the sebum are not unique to people with Parkinson's, but they are differently expressed," says Barran, a professor of mass spectrometry at the University of Manchester. "So this test we're developing now is not a black-and-white, do-you-have-something kind of test, but rather how much of these compounds do you have compared to other people and other compounds." The team identified over a dozen compounds that were present in the sebum of Parkinson's patients in much larger amounts than the control group.
Using only the GC-MS and a sebum swab test, Barran's team can now correctly identify Parkinson's in nine out of 10 patients – a much quicker and more accurate way to diagnose than what clinicians do now.
"At the moment, a clinical diagnosis is based on the patient's physical symptoms," Barran says, and determining whether a patient has Parkinson's is often a long and drawn-out process of elimination. "Doctors might say that a group of symptoms looks like Parkinson's, but there are other reasons people might have those symptoms, and it might take another year before they're certain," Barran says. "Some of those symptoms are just signs of aging, and other symptoms like tremor are present in recovering alcoholics or people with other kinds of dementia." People under the age of 40 with Parkinson's symptoms, who present with stiff arms, are often misdiagnosed with carpal tunnel syndrome, she adds.
Additionally, by the time physical symptoms are present, Parkinson's patients have already lost a substantial amount of dopamine receptors – about sixty percent -- in the brain's basal ganglia. Getting a diagnosis before physical symptoms appear would mean earlier interventions that could prevent dopamine loss and preserve regular movement, Barran says.
"Early diagnosis is good if it means there's a chance of early intervention," says Barran. "It stops the process of dopamine loss, which means that motor symptoms potentially will not happen, or the onset of symptoms will be substantially delayed." Barran's team is in the processing of streamlining the sebum test so that definitive results will be ready in just two minutes.
"What we're doing right now will be a very inexpensive test, a rapid-screen test, and that will encourage people to self-sample and test at home," says Barran. In addition to diagnosing Parkinson's, she says, this test could also be potentially useful to determine if medications were at a therapeutic dose in people who have the disease, since the odor is strongest in people whose symptoms are least controlled by medication.
"When symptoms are under control, the odor is lower," Barran says. "Potentially this would allow patients and clinicians to see whether their symptoms are being managed properly with medication, or perhaps if they're being overmedicated." Hypothetically, patients could also use the test to determine if interventions like diet and exercise are effective at keeping Parkinson's controlled.
"We hope within the next two to five years we will have a test available."
Barran is now running another clinical trial – one that determines whether they can diagnose at an earlier stage and whether they can identify a difference in sebum samples between different forms of Parkinson's or diseases that have Parkinson's-like symptoms, such as Lewy Body Dementia.
"Within the next one to two years, we hope to be running a trial in the Manchester area for those people who do not have motor symptoms but are at risk for developing dementia due to symptoms like loss of smell and sleep difficulty," Barran had said in 2019. "If we can establish that, we can roll out a test that determines if you have Parkinson's or not with those first pre-motor symptoms, and then at what stage. We hope within the next two to five years we will have a test available."
In a 2022 study, published in the American Chemical Society, researchers used mass spectrometry to analyze sebum from skin swabs for the presence of the specific molecules. They found that some specific molecules are present only in people who have Parkinson’s. Now they hope that the same method can be used in regular diagnostic labs. The test, many years in the making, is inching its way to the clinic.
"We would likely first give this test to people who are at risk due to a genetic predisposition, or who are at risk based on prodomal symptoms, like people who suffer from a REM sleep disorder who have a 50 to 70 percent chance of developing Parkinson's within a ten year period," Barran says. "Those would be people who would benefit from early therapeutic intervention. For the normal population, it isn't beneficial at the moment to know until we have therapeutic interventions that can be useful."
Milne's husband, Les, passed away from complications of Parkinson's Disease in 2015. But thanks to him and the dedication of his wife, Joy, science may have found a way to someday prolong the lives of others with this devastating disease. Sometimes she can smell people who have Parkinson’s while in the supermarket or walking down the street but has been told by medical ethicists she cannot tell them, Milne said in an interview with the Guardian. But once the test becomes available in the clinics, it will do the job for her.
[Ed. Note: A older version of this hit article originally ran on September 3, 2019.]