AI and you: Is the promise of personalized nutrition apps worth the hype?
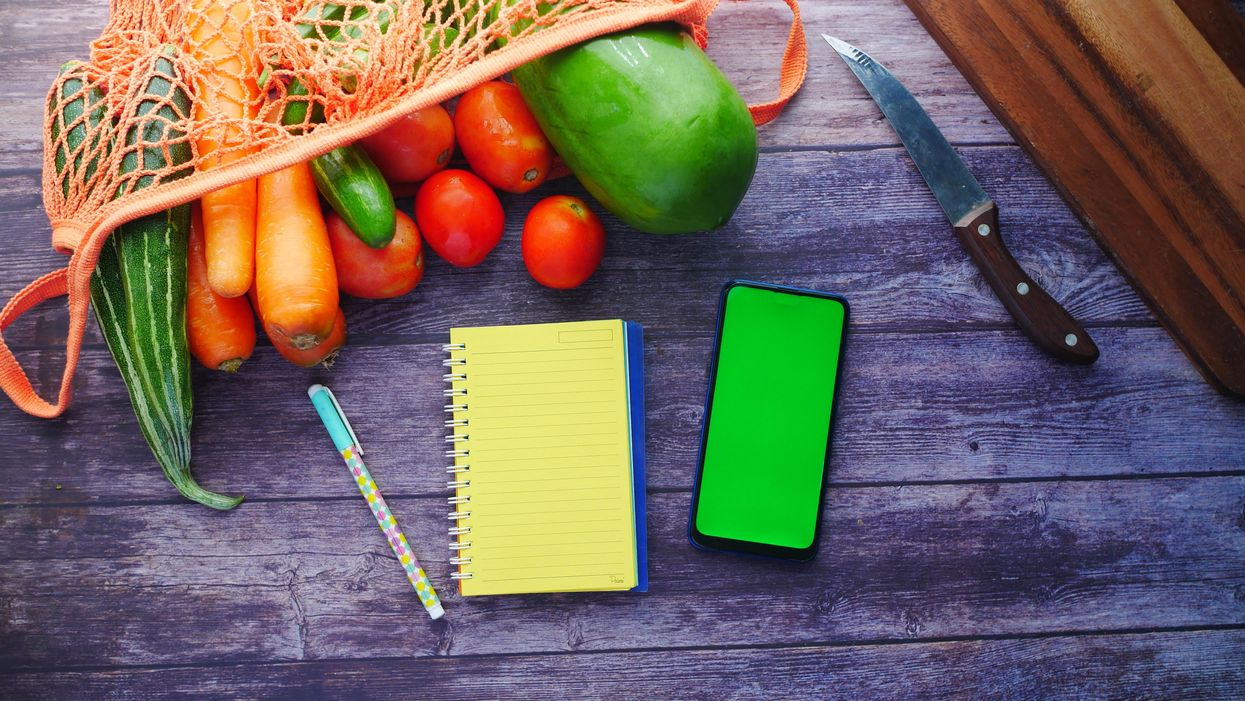
Personalized nutrition apps could provide valuable data to people trying to eat healthier, though more research must be done to show effectiveness.
As a type 2 diabetic, Michael Snyder has long been interested in how blood sugar levels vary from one person to another in response to the same food, and whether a more personalized approach to nutrition could help tackle the rapidly cascading levels of diabetes and obesity in much of the western world.
Eight years ago, Snyder, who directs the Center for Genomics and Personalized Medicine at Stanford University, decided to put his theories to the test. In the 2000s continuous glucose monitoring, or CGM, had begun to revolutionize the lives of diabetics, both type 1 and type 2. Using spherical sensors which sit on the upper arm or abdomen – with tiny wires that pierce the skin – the technology allowed patients to gain real-time updates on their blood sugar levels, transmitted directly to their phone.
It gave Snyder an idea for his research at Stanford. Applying the same technology to a group of apparently healthy people, and looking for ‘spikes’ or sudden surges in blood sugar known as hyperglycemia, could provide a means of observing how their bodies reacted to an array of foods.
“We discovered that different foods spike people differently,” he says. “Some people spike to pasta, others to bread, others to bananas, and so on. It’s very personalized and our feeling was that building programs around these devices could be extremely powerful for better managing people’s glucose.”
Unbeknown to Snyder at the time, thousands of miles away, a group of Israeli scientists at the Weizmann Institute of Science were doing exactly the same experiments. In 2015, they published a landmark paper which used CGM to track the blood sugar levels of 800 people over several days, showing that the biological response to identical foods can vary wildly. Like Snyder, they theorized that giving people a greater understanding of their own glucose responses, so they spend more time in the normal range, may reduce the prevalence of type 2 diabetes.
The commercial potential of such apps is clear, but the underlying science continues to generate intriguing findings.
“At the moment 33 percent of the U.S. population is pre-diabetic, and 70 percent of those pre-diabetics will become diabetic,” says Snyder. “Those numbers are going up, so it’s pretty clear we need to do something about it.”
Fast forward to 2022,and both teams have converted their ideas into subscription-based dietary apps which use artificial intelligence to offer data-informed nutritional and lifestyle recommendations. Snyder’s spinoff, January AI, combines CGM information with heart rate, sleep, and activity data to advise on foods to avoid and the best times to exercise. DayTwo–a start-up which utilizes the findings of Weizmann Institute of Science–obtains microbiome information by sequencing stool samples, and combines this with blood glucose data to rate ‘good’ and ‘bad’ foods for a particular person.
“CGMs can be used to devise personalized diets,” says Eran Elinav, an immunology professor and microbiota researcher at the Weizmann Institute of Science in addition to serving as a scientific consultant for DayTwo. “However, this process can be cumbersome. Therefore, in our lab we created an algorithm, based on data acquired from a big cohort of people, which can accurately predict post-meal glucose responses on a personal basis.”
The commercial potential of such apps is clear. DayTwo, who market their product to corporate employers and health insurers rather than individual consumers, recently raised $37 million in funding. But the underlying science continues to generate intriguing findings.
Last year, Elinav and colleagues published a study on 225 individuals with pre-diabetes which found that they achieved better blood sugar control when they followed a personalized diet based on DayTwo’s recommendations, compared to a Mediterranean diet. The journal Cell just released a new paper from Snyder’s group which shows that different types of fibre benefit people in different ways.
“The idea is you hear different fibres are good for you,” says Snyder. “But if you look at fibres they’re all over the map—it’s like saying all animals are the same. The responses are very individual. For a lot of people [a type of fibre called] arabinoxylan clearly reduced cholesterol while the fibre inulin had no effect. But in some people, it was the complete opposite.”
Eight years ago, Stanford's Michael Snyder began studying how continuous glucose monitors could be used by patients to gain real-time updates on their blood sugar levels, transmitted directly to their phone.
The Snyder Lab, Stanford Medicine
Because of studies like these, interest in precision nutrition approaches has exploded in recent years. In January, the National Institutes of Health announced that they are spending $170 million on a five year, multi-center initiative which aims to develop algorithms based on a whole range of data sources from blood sugar to sleep, exercise, stress, microbiome and even genomic information which can help predict which diets are most suitable for a particular individual.
“There's so many different factors which influence what you put into your mouth but also what happens to different types of nutrients and how that ultimately affects your health, which means you can’t have a one-size-fits-all set of nutritional guidelines for everyone,” says Bruce Y. Lee, professor of health policy and management at the City University of New York Graduate School of Public Health.
With the falling costs of genomic sequencing, other precision nutrition clinical trials are choosing to look at whether our genomes alone can yield key information about what our diets should look like, an emerging field of research known as nutrigenomics.
The ASPIRE-DNA clinical trial at Imperial College London is aiming to see whether particular genetic variants can be used to classify individuals into two groups, those who are more glucose sensitive to fat and those who are more sensitive to carbohydrates. By following a tailored diet based on these sensitivities, the trial aims to see whether it can prevent people with pre-diabetes from developing the disease.
But while much hope is riding on these trials, even precision nutrition advocates caution that the field remains in the very earliest of stages. Lars-Oliver Klotz, professor of nutrigenomics at Friedrich-Schiller-University in Jena, Germany, says that while the overall goal is to identify means of avoiding nutrition-related diseases, genomic data alone is unlikely to be sufficient to prevent obesity and type 2 diabetes.
“Genome data is rather simple to acquire these days as sequencing techniques have dramatically advanced in recent years,” he says. “However, the predictive value of just genome sequencing is too low in the case of obesity and prediabetes.”
Others say that while genomic data can yield useful information in terms of how different people metabolize different types of fat and specific nutrients such as B vitamins, there is a need for more research before it can be utilized in an algorithm for making dietary recommendations.
“I think it’s a little early,” says Eileen Gibney, a professor at University College Dublin. “We’ve identified a limited number of gene-nutrient interactions so far, but we need more randomized control trials of people with different genetic profiles on the same diet, to see whether they respond differently, and if that can be explained by their genetic differences.”
Some start-ups have already come unstuck for promising too much, or pushing recommendations which are not based on scientifically rigorous trials. The world of precision nutrition apps was dubbed a ‘Wild West’ by some commentators after the founders of uBiome – a start-up which offered nutritional recommendations based on information obtained from sequencing stool samples –were charged with fraud last year. The weight-loss app Noom, which was valued at $3.7 billion in May 2021, has been criticized on Twitter by a number of users who claimed that its recommendations have led to them developed eating disorders.
With precision nutrition apps marketing their technology at healthy individuals, question marks have also been raised about the value which can be gained through non-diabetics monitoring their blood sugar through CGM. While some small studies have found that wearing a CGM can make overweight or obese individuals more motivated to exercise, there is still a lack of conclusive evidence showing that this translates to improved health.
However, independent researchers remain intrigued by the technology, and say that the wealth of data generated through such apps could be used to help further stratify the different types of people who become at risk of developing type 2 diabetes.
“CGM not only enables a longer sampling time for capturing glucose levels, but will also capture lifestyle factors,” says Robert Wagner, a diabetes researcher at University Hospital Düsseldorf. “It is probable that it can be used to identify many clusters of prediabetic metabolism and predict the risk of diabetes and its complications, but maybe also specific cardiometabolic risk constellations. However, we still don’t know which forms of diabetes can be prevented by such approaches and how feasible and long-lasting such self-feedback dietary modifications are.”
Snyder himself has now been wearing a CGM for eight years, and he credits the insights it provides with helping him to manage his own diabetes. “My CGM still gives me novel insights into what foods and behaviors affect my glucose levels,” he says.
He is now looking to run clinical trials with his group at Stanford to see whether following a precision nutrition approach based on CGM and microbiome data, combined with other health information, can be used to reverse signs of pre-diabetes. If it proves successful, January AI may look to incorporate microbiome data in future.
“Ultimately, what I want to do is be able take people’s poop samples, maybe a blood draw, and say, ‘Alright, based on these parameters, this is what I think is going to spike you,’ and then have a CGM to test that out,” he says. “Getting very predictive about this, so right from the get go, you can have people better manage their health and then use the glucose monitor to help follow that.”
After spaceflight record, NASA looks to protect astronauts on even longer trips
NASA astronaut Frank Rubio floats by the International Space Station’s “window to the world.” Yesterday, he returned from the longest single spaceflight by a U.S. astronaut on record - over one year. Exploring deep space will require even longer missions.
At T-minus six seconds, the main engines of the Atlantis Space Shuttle ignited, rattling its capsule “like a skyscraper in an earthquake,” according to astronaut Tom Jones, describing the 1988 launch. As the rocket lifted off and accelerated to three times the force of Earth's gravity, “It felt as if two of my friends were standing on my chest and wouldn’t get off.” But when Atlantis reached orbit, the main engines cut off, and the astronauts were suddenly weightless.
Since 1961, NASA has sent hundreds of astronauts into space while working to making their voyages safer and smoother. Yet, challenges remain. Weightlessness may look amusing when watched from Earth, but it has myriad effects on cognition, movement and other functions. When missions to space stretch to six months or longer, microgravity can impact astronauts’ health and performance, making it more difficult to operate their spacecraft.
Yesterday, NASA astronaut Frank Rubio returned to Earth after over one year, the longest single spaceflight for a U.S. astronaut. But this is just the start; longer and more complex missions into deep space loom ahead, from returning to the moon in 2025 to eventually sending humans to Mars. To ensure that these missions succeed, NASA is increasing efforts to study the biological effects and prevent harm.
The dangers of microgravity are real
A NASA report published in 2016 details a long list of incidents and near-misses caused – at least partly – by space-induced changes in astronauts’ vision and coordination. These issues make it harder to move with precision and to judge distance and velocity.
According to the report, in 1997, a resupply ship collided with the Mir space station, possibly because a crew member bumped into the commander during the final docking maneuver. This mishap caused significant damage to the space station.
Returns to Earth suffered from problems, too. The same report notes that touchdown speeds during the first 100 space shuttle landings were “outside acceptable limits. The fastest landing on record – 224 knots (258 miles) per hour – was linked to the commander’s momentary spatial disorientation.” Earlier, each of the six Apollo crews that landed on the moon had difficulty recognizing moon landmarks and estimating distances. For example, Apollo 15 landed in an unplanned area, ultimately straddling the rim of a five-foot deep crater on the moon, harming one of its engines.
Spaceflight causes unique stresses on astronauts’ brains and central nervous systems. NASA is working to reduce these harmful effects.
NASA
Space messes up your brain
In space, astronauts face the challenges of microgravity, ionizing radiation, social isolation, high workloads, altered circadian rhythms, monotony, confined living quarters and a high-risk environment. Among these issues, microgravity is one of the most consequential in terms of physiological changes. It changes the brain’s structure and its functioning, which can hurt astronauts’ performance.
The brain shifts upwards within the skull, displacing the cerebrospinal fluid, which reduces the brain’s cushioning. Essentially, the brain becomes crowded inside the skull like a pair of too-tight shoes.
That’s partly because of how being in space alters blood flow. On Earth, gravity pulls our blood and other internal fluids toward our feet, but our circulatory valves ensure that the fluids are evenly distributed throughout the body. In space, there’s not enough gravity to pull the fluids down, and they shift up, says Rachael D. Seidler, a physiologist specializing in spaceflight at the University of Florida and principal investigator on many space-related studies. The head swells and legs appear thinner, causing what astronauts call “puffy face chicken legs.”
“The brain changes at the structural and functional level,” says Steven Jillings, equilibrium and aerospace researcher at the University of Antwerp in Belgium. “The brain shifts upwards within the skull,” displacing the cerebrospinal fluid, which reduces the brain’s cushioning. Essentially, the brain becomes crowded inside the skull like a pair of too-tight shoes. Some of the displaced cerebrospinal fluid goes into cavities within the brain, called ventricles, enlarging them. “The remaining fluids pool near the chest and heart,” explains Jillings. After 12 consecutive months in space, one astronaut had a ventricle that was 25 percent larger than before the mission.
Some changes reverse themselves while others persist for a while. An example of a longer-lasting problem is spaceflight-induced neuro-ocular syndrome, which results in near-sightedness and pressure inside the skull. A study of approximately 300 astronauts shows near-sightedness affects about 60 percent of astronauts after long missions on the International Space Station (ISS) and more than 25 percent after spaceflights of only a few weeks.
Another long-term change could be the decreased ability of cerebrospinal fluid to clear waste products from the brain, Seidler says. That’s because compressing the brain also compresses its waste-removing glymphatic pathways, resulting in inflammation, vulnerability to injuries and worsening its overall health.
The effects of long space missions were best demonstrated on astronaut twins Scott and Mark Kelly. This NASA Twins Study showed multiple, perhaps permanent, changes in Scott after his 340-day mission aboard the ISS, compared to Mark, who remained on Earth. The differences included declines in Scott’s speed, accuracy and cognitive abilities that persisted longer than six months after returning to Earth in March 2016.
By the end of 2020, Scott’s cognitive abilities improved, but structural and physiological changes to his eyes still remained, he said in a BBC interview.
“It seems clear that the upward shift of the brain and compression of the surrounding tissues with ventricular expansion might not be a good thing,” Seidler says. “But, at this point, the long-term consequences to brain health and human performance are not really known.”
NASA astronaut Kate Rubins conducts a session for the Neuromapping investigation.
NASA
Staying sharp in space
To investigate how prolonged space travel affects the brain, NASA launched a new initiative called the Complement of Integrated Protocols for Human Exploration Research (CIPHER). “CIPHER investigates how long-duration spaceflight affects both brain structure and function,” says neurobehavioral scientist Mathias Basner at the University of Pennsylvania, a principal investigator for several NASA studies. “Through it, we can find out how the brain adapts to the spaceflight environment and how certain brain regions (behave) differently after – relative to before – the mission.”
To do this, he says, “Astronauts will perform NASA’s cognition test battery before, during and after six- to 12-month missions, and will also perform the same test battery in an MRI scanner before and after the mission. We have to make sure we better understand the functional consequences of spaceflight on the human brain before we can send humans safely to the moon and, especially, to Mars.”
As we go deeper into space, astronauts cognitive and physical functions will be even more important. “A trip to Mars will take about one year…and will introduce long communication delays,” Seidler says. “If you are on that mission and have a problem, it may take eight to 10 minutes for your message to reach mission control, and another eight to 10 minutes for the response to get back to you.” In an emergency situation, that may be too late for the response to matter.
“On a mission to Mars, astronauts will be exposed to stressors for unprecedented amounts of time,” Basner says. To counter them, NASA is considering the continuous use of artificial gravity during the journey, and Seidler is studying whether artificial gravity can reduce the harmful effects of microgravity. Some scientists are looking at precision brain stimulation as a way to improve memory and reduce anxiety due to prolonged exposure to radiation in space.
Other scientists are exploring how to protect neural stem cells (which create brain cells) from radiation damage, developing drugs to repair damaged brain cells and protect cells from radiation.
To boldly go where no astronauts have gone before, they must have optimal reflexes, vision and decision-making. In the era of deep space exploration, the brain—without a doubt—is the final frontier.
Additionally, NASA is scrutinizing each aspect of the mission, including astronaut exercise, nutrition and intellectual engagement. “We need to give astronauts meaningful work. We need to stimulate their sensory, cognitive and other systems appropriately,” Basner says, especially given their extreme confinement and isolation. The scientific experiments performed on the ISS – like studying how microgravity affects the ability of tissue to regenerate is a good example.
“We need to keep them engaged socially, too,” he continues. The ISS crew, for example, regularly broadcasts from space and answers prerecorded questions from students on Earth, and can engage with social media in real time. And, despite tight quarters, NASA is ensuring the crew capsule and living quarters on the moon or Mars include private space, which is critical for good mental health.
Exploring deep space builds on a foundation that began when astronauts first left the planet. With each mission, scientists learn more about spaceflight effects on astronauts’ bodies. NASA will be using these lessons to succeed with its plans to build science stations on the moon and, eventually, Mars.
“Through internally and externally led research, investigations implemented in space and in spaceflight simulations on Earth, we are striving to reduce the likelihood and potential impacts of neurostructural changes in future, extended spaceflight,” summarizes NASA scientist Alexandra Whitmire. To boldly go where no astronauts have gone before, they must have optimal reflexes, vision and decision-making. In the era of deep space exploration, the brain—without a doubt—is the final frontier.
A newly discovered brain cell may lead to better treatments for cognitive disorders
Swiss researchers have found a type of brain cell that appears to be a hybrid of the two other main types — and it could lead to new treatments for brain disorders.
Swiss researchers have discovered a third type of brain cell that appears to be a hybrid of the two other primary types — and it could lead to new treatments for many brain disorders.
The challenge: Most of the cells in the brain are either neurons or glial cells. While neurons use electrical and chemical signals to send messages to one another across small gaps called synapses, glial cells exist to support and protect neurons.
Astrocytes are a type of glial cell found near synapses. This close proximity to the place where brain signals are sent and received has led researchers to suspect that astrocytes might play an active role in the transmission of information inside the brain — a.k.a. “neurotransmission” — but no one has been able to prove the theory.
A new brain cell: Researchers at the Wyss Center for Bio and Neuroengineering and the University of Lausanne believe they’ve definitively proven that some astrocytes do actively participate in neurotransmission, making them a sort of hybrid of neurons and glial cells.
According to the researchers, this third type of brain cell, which they call a “glutamatergic astrocyte,” could offer a way to treat Alzheimer’s, Parkinson’s, and other disorders of the nervous system.
“Its discovery opens up immense research prospects,” said study co-director Andrea Volterra.
The study: Neurotransmission starts with a neuron releasing a chemical called a neurotransmitter, so the first thing the researchers did in their study was look at whether astrocytes can release the main neurotransmitter used by neurons: glutamate.
By analyzing astrocytes taken from the brains of mice, they discovered that certain astrocytes in the brain’s hippocampus did include the “molecular machinery” needed to excrete glutamate. They found evidence of the same machinery when they looked at datasets of human glial cells.
Finally, to demonstrate that these hybrid cells are actually playing a role in brain signaling, the researchers suppressed their ability to secrete glutamate in the brains of mice. This caused the rodents to experience memory problems.
“Our next studies will explore the potential protective role of this type of cell against memory impairment in Alzheimer’s disease, as well as its role in other regions and pathologies than those explored here,” said Andrea Volterra, University of Lausanne.
But why? The researchers aren’t sure why the brain needs glutamatergic astrocytes when it already has neurons, but Volterra suspects the hybrid brain cells may help with the distribution of signals — a single astrocyte can be in contact with thousands of synapses.
“Often, we have neuronal information that needs to spread to larger ensembles, and neurons are not very good for the coordination of this,” researcher Ludovic Telley told New Scientist.
Looking ahead: More research is needed to see how the new brain cell functions in people, but the discovery that it plays a role in memory in mice suggests it might be a worthwhile target for Alzheimer’s disease treatments.
The researchers also found evidence during their study that the cell might play a role in brain circuits linked to seizures and voluntary movements, meaning it’s also a new lead in the hunt for better epilepsy and Parkinson’s treatments.
“Our next studies will explore the potential protective role of this type of cell against memory impairment in Alzheimer’s disease, as well as its role in other regions and pathologies than those explored here,” said Volterra.