How Bacteria-Killing Viruses May Save Us From Antibiotic Resistance
Dr. Adalja is focused on emerging infectious disease, pandemic preparedness, and biosecurity. He has served on US government panels tasked with developing guidelines for the treatment of plague, botulism, and anthrax in mass casualty settings and the system of care for infectious disease emergencies, and as an external advisor to the New York City Health and Hospital Emergency Management Highly Infectious Disease training program, as well as on a FEMA working group on nuclear disaster recovery. Dr. Adalja is an Associate Editor of the journal Health Security. He was a coeditor of the volume Global Catastrophic Biological Risks, a contributing author for the Handbook of Bioterrorism and Disaster Medicine, the Emergency Medicine CorePendium, Clinical Microbiology Made Ridiculously Simple, UpToDate's section on biological terrorism, and a NATO volume on bioterrorism. He has also published in such journals as the New England Journal of Medicine, the Journal of Infectious Diseases, Clinical Infectious Diseases, Emerging Infectious Diseases, and the Annals of Emergency Medicine. He is a board-certified physician in internal medicine, emergency medicine, infectious diseases, and critical care medicine. Follow him on Twitter: @AmeshAA
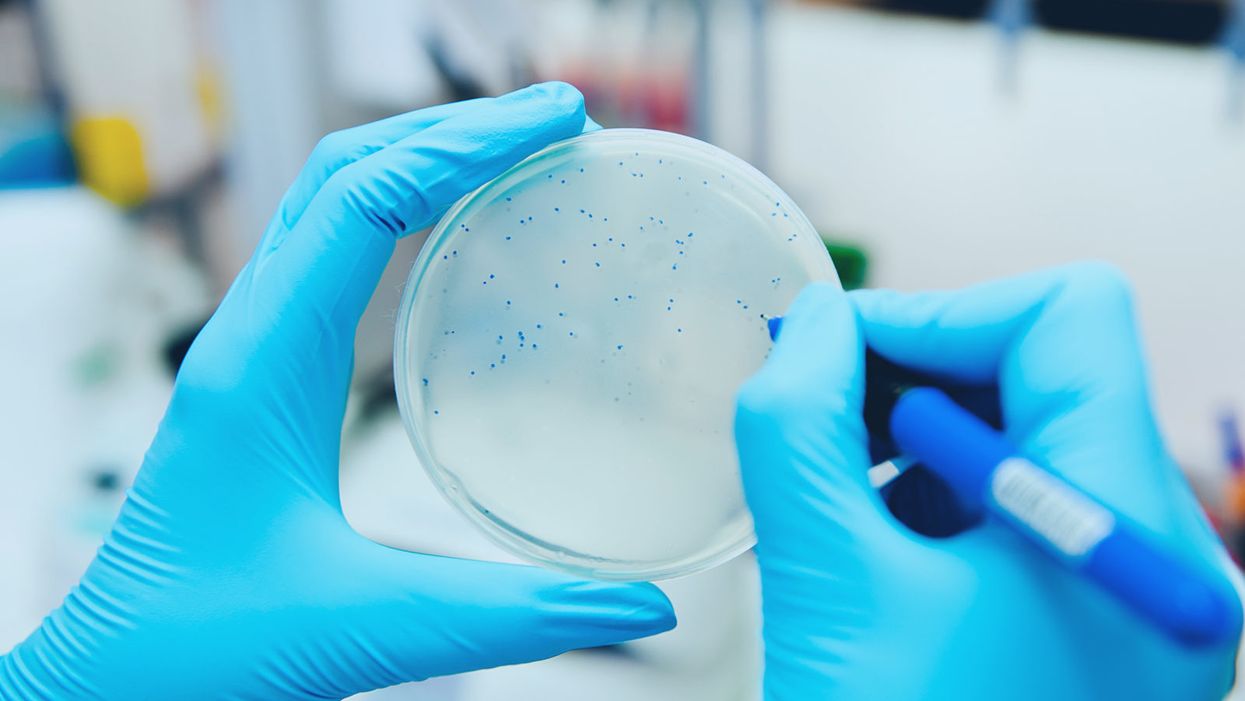
Hand-counting bacteriophage plaques during a titer test.
In my hometown of Pittsburgh, it is not uncommon to read about cutting-edge medical breakthroughs, because Pittsburgh is the home of many innovations in medical science, from the polio vaccine to pioneering organ transplantation. However, medical headlines from Pittsburgh last November weren't heralding a new discovery for once. They were carrying a plea—for a virus.
Phages are weapons of bacterial destruction, but despite recognition of their therapeutic potential for over 100 years, there are zero phage products commercially available to medicine in the United States.
Specifically, a bacteria-killing virus that could attack and control a certain highly drug-resistant bacterial infection ravaging the newly transplanted lungs of a 25-year-old woman named Mallory Smith. The culprit bacteria, Burkholderia cepacia, is a notoriously vicious bacterium that preys on patients with cystic fibrosis who, throughout their life, are exposed to course after course of antibiotics, often fostering a population of highly resistant bacteria that can become too formidable for modern medicine to combat.
What Smith and her physicians desperately needed was a tool that would move beyond failed courses of antibiotics. What they sought was called a bacteriophage. These are naturally occurring ubiquitous viruses that target not humans, but bacteria. The world literally teems with "phages" and one cannot take a bite or drink of anything without encountering them. These weapons of bacterial destruction are exquisitely evolved to target bacteria and, as such, are not harmful to humans. However, despite recognition of their therapeutic potential for over 100 years, there are zero bacteriophage products commercially available to medicine in the United States, at a time when antibiotic resistance is arguably our most pressing public health crisis. Just this week, a new study was published in the Proceedings of the National Academy of Sciences detailing the global scope of the problem.
Why Were These Promising Tools Forgotten?
Phages weren't always relegated to this status. In fact, in the early 20th century phages could be found on American drug store shelves and were used for a variety of ailments. However, the path-breaking discovery and development of antimicrobials agents such as the sulfa drugs and, later the antibiotic penicillin, supplanted the world of phage therapeutics in the United States and many other places.
Fortunately, phage therapy never fully disappeared, and research and clinical use continued in Eastern European nations such as Georgia and Poland.
The antibiotic age revolutionized medicine in a way that arguably no other innovation has. Not only did antibiotics tame many once-deadly infectious diseases, but they made much of modern medicine – from cancer chemotherapy to organ transplantation to joint replacement – possible. Antibiotics, unlike the exquisitely evolved bacteriophage, possessed a broader spectrum of activity and were active against a range of bacteria. This non-specificity facilitated antibiotic use without the need for a specific diagnosis. A physician does not need to know the specific bacterial genus and species causing, for example, a skin infection or pneumonia, but can select an antibiotic that covers the likely culprits and use it empirically, fully expecting the infection to be controlled. Unfortunately, this non-specificity engendered the overuse of antibiotics whose consequences we are now suffering. A bacteriophage, on the other hand, will work against one specific bacterial species and is evolved for just that role.
Phages to the Rescue
As the march of antibiotic resistance has predictably continued since the dawn of the antibiotic age, the prospect of resurrecting phage therapy has been increasingly viewed as one solution. Fortunately, phage therapy never fully disappeared, and research and clinical use continued in Eastern European nations such as Georgia and Poland. However, much of that experience has remained opaque to the medical community at large and questions about dosage, toxicity, efficacy, and method of delivery left many questions without full answers.
Though real questions remained regarding phage use, dire circumstances of prolific antibiotic resistance necessitated their use in the U.S. in two prominent instances involving life-threatening infections. The first case involved an Acinetobacter baumanii infection of the pancreas in a San Diego man in which phages were administered intravenously in 2016. The other case, also in 2016, involved the instillation of phages, fished out of a pond, into the chest cavity of man with a Pseudmonas aeruginosa infection of a prosthetic graft of the aorta. Both cases were successful and were what fueled the Pittsburgh-based plea for Burkholderia phages.
The phages you begin with may not be the ones you end up with, as Darwinian evolutionary pressures will alter the phage in order to keep up with the ongoing evolution of its bacterial target.
How Phages Differ from Other Medical Products
It might seem surprising that in light of the urgent need for new treatments for drug-resistant infections, the pharmaceutical armamentarium is not teeming with phages like a backyard pond. However, phages have been difficult to fit into the current regulatory framework that operates in most developed countries such as the U.S. because of their unique characteristics.
Phages are not one homogenous product like a tablet of penicillin, but a cocktail of viruses that change and evolve as they replicate. The phages you begin with may not be the ones you end up with, as Darwinian evolutionary pressures will alter the phage in order to keep up with the ongoing evolution of its bacterial target. The cocktail may not just contain one specific phage, but a range of phages that all target some specific bacteria in order to increase efficacy. These phage cocktails might also need adjusting to keep pace with bacterial resistance. Additionally, the concentration of phage in a human body after administration is not so easy to predict as phage numbers will rise and fall based on the number of target bacteria that are present.
All of these characteristics make phages very unique when viewed through a regulatory lens, and necessitate the creation of new methods to evaluate them, given that regulatory approval is required. Using phages in the U.S. now requires FDA permission through an investigational new drug application, which can be expedited during an emergency situation. FDA scientists are actively involved in understanding the best means to evaluate bacteriophage therapy and several companies are in early-stage development, though no major clinical trials in the U.S. are currently underway.
One FDA-approved application of phages has seen them used on food products at delis and even in slaughterhouses to diminish the quantity of bacteria on certain meat products.
Would That Humans Were As Lucky As Bologna
Because of the regulatory difficulties with human-use approval, some phage companies have taken another route to develop phage products: food safety. Food safety is a major public health endeavor, and keeping food that people consume safe from E.coli, Listeria, and Salmonella, for example, are rightfully major priorities of industry. One FDA-approved application of phages has seen them used on food products at delis and even in slaughterhouses to diminish the quantity of bacteria on certain meat products.
This use, unlike that for human therapeutic purposes, has found success with regulators: phages, not surprisingly, have been granted the "generally regarded as safe (GRAS)" designation.
A Phage Directory
Tragically Mallory Smith succumbed to her infection despite getting a dose of phages culled from sludge in the Philippines and Fiji. However, her death and last-minute crusade to obtain phages has prompted the call for a phage directory. This directory could catalog the various phages being studied and the particular bacteria they target. Such a searchable index will facilitate the rapid identification and – hopefully – delivery of phages to patients.
If phage therapy is to move from a last-ditch emergency measure to a routine tool for infectious disease physicians, it will be essential that the hurdles they face are eliminated.
Moving Beyond Antibiotics
As we move increasingly toward a post-antibiotic age in infectious disease, moving outside of the traditional paradigm of broad-spectrum antibiotics to non-traditional therapeutics such as bacteriophages and other novel products will become increasingly necessary. Already, clinical trials are underway in various populations, including a major trial in European burn patients.
It is important to understand that there are important scientific and therapeutic questions regarding dose, route of administration and other related questions that need to be addressed before phage use becomes more routine, and it is only through clinical trials conducted with the hope of eventual commercialization that these answers will be found. If phage therapy is to move from a last-ditch emergency measure to a routine tool for infectious disease physicians, it will be essential that the hurdles they face are eliminated.
Dr. Adalja is focused on emerging infectious disease, pandemic preparedness, and biosecurity. He has served on US government panels tasked with developing guidelines for the treatment of plague, botulism, and anthrax in mass casualty settings and the system of care for infectious disease emergencies, and as an external advisor to the New York City Health and Hospital Emergency Management Highly Infectious Disease training program, as well as on a FEMA working group on nuclear disaster recovery. Dr. Adalja is an Associate Editor of the journal Health Security. He was a coeditor of the volume Global Catastrophic Biological Risks, a contributing author for the Handbook of Bioterrorism and Disaster Medicine, the Emergency Medicine CorePendium, Clinical Microbiology Made Ridiculously Simple, UpToDate's section on biological terrorism, and a NATO volume on bioterrorism. He has also published in such journals as the New England Journal of Medicine, the Journal of Infectious Diseases, Clinical Infectious Diseases, Emerging Infectious Diseases, and the Annals of Emergency Medicine. He is a board-certified physician in internal medicine, emergency medicine, infectious diseases, and critical care medicine. Follow him on Twitter: @AmeshAA
A new type of cancer therapy is shrinking deadly brain tumors with just one treatment
MRI scans after a new kind of immunotherapy for brain cancer show remarkable progress in one patient just days after the first treatment.
Few cancers are deadlier than glioblastomas—aggressive and lethal tumors that originate in the brain or spinal cord. Five years after diagnosis, less than five percent of glioblastoma patients are still alive—and more often, glioblastoma patients live just 14 months on average after receiving a diagnosis.
But an ongoing clinical trial at Mass General Cancer Center is giving new hope to glioblastoma patients and their families. The trial, called INCIPIENT, is meant to evaluate the effects of a special type of immune cell, called CAR-T cells, on patients with recurrent glioblastoma.
How CAR-T cell therapy works
CAR-T cell therapy is a type of cancer treatment called immunotherapy, where doctors modify a patient’s own immune system specifically to find and destroy cancer cells. In CAR-T cell therapy, doctors extract the patient’s T-cells, which are immune system cells that help fight off disease—particularly cancer. These T-cells are harvested from the patient and then genetically modified in a lab to produce proteins on their surface called chimeric antigen receptors (thus becoming CAR-T cells), which makes them able to bind to a specific protein on the patient’s cancer cells. Once modified, these CAR-T cells are grown in the lab for several weeks so that they can multiply into an army of millions. When enough cells have been grown, these super-charged T-cells are infused back into the patient where they can then seek out cancer cells, bind to them, and destroy them. CAR-T cell therapies have been approved by the US Food and Drug Administration (FDA) to treat certain types of lymphomas and leukemias, as well as multiple myeloma, but haven’t been approved to treat glioblastomas—yet.
CAR-T cell therapies don’t always work against solid tumors, such as glioblastomas. Because solid tumors contain different kinds of cancer cells, some cells can evade the immune system’s detection even after CAR-T cell therapy, according to a press release from Massachusetts General Hospital. For the INCIPIENT trial, researchers modified the CAR-T cells even further in hopes of making them more effective against solid tumors. These second-generation CAR-T cells (called CARv3-TEAM-E T cells) contain special antibodies that attack EFGR, a protein expressed in the majority of glioblastoma tumors. Unlike other CAR-T cell therapies, these particular CAR-T cells were designed to be directly injected into the patient’s brain.
The INCIPIENT trial results
The INCIPIENT trial involved three patients who were enrolled in the study between March and July 2023. All three patients—a 72-year-old man, a 74-year-old man, and a 57-year-old woman—were treated with chemo and radiation and enrolled in the trial with CAR-T cells after their glioblastoma tumors came back.
The results, which were published earlier this year in the New England Journal of Medicine (NEJM), were called “rapid” and “dramatic” by doctors involved in the trial. After just a single infusion of the CAR-T cells, each patient experienced a significant reduction in their tumor sizes. Just two days after receiving the infusion, the glioblastoma tumor of the 72-year-old man decreased by nearly twenty percent. Just two months later the tumor had shrunk by an astonishing 60 percent, and the change was maintained for more than six months. The most dramatic result was in the 57-year-old female patient, whose tumor shrank nearly completely after just one infusion of the CAR-T cells.
The results of the INCIPIENT trial were unexpected and astonishing—but unfortunately, they were also temporary. For all three patients, the tumors eventually began to grow back regardless of the CAR-T cell infusions. According to the press release from MGH, the medical team is now considering treating each patient with multiple infusions or prefacing each treatment with chemotherapy to prolong the response.
While there is still “more to do,” says co-author of the study neuro-oncologist Dr. Elizabeth Gerstner, the results are still promising. If nothing else, these second-generation CAR-T cell infusions may someday be able to give patients more time than traditional treatments would allow.
“These results are exciting but they are also just the beginning,” says Dr. Marcela Maus, a doctor and professor of medicine at Mass General who was involved in the clinical trial. “They tell us that we are on the right track in pursuing a therapy that has the potential to change the outlook for this intractable disease.”
A recent study in The Lancet Oncology showed that AI found 20 percent more cancers on mammogram screens than radiologists alone.
Since the early 2000s, AI systems have eliminated more than 1.7 million jobs, and that number will only increase as AI improves. Some research estimates that by 2025, AI will eliminate more than 85 million jobs.
But for all the talk about job security, AI is also proving to be a powerful tool in healthcare—specifically, cancer detection. One recently published study has shown that, remarkably, artificial intelligence was able to detect 20 percent more cancers in imaging scans than radiologists alone.
Published in The Lancet Oncology, the study analyzed the scans of 80,000 Swedish women with a moderate hereditary risk of breast cancer who had undergone a mammogram between April 2021 and July 2022. Half of these scans were read by AI and then a radiologist to double-check the findings. The second group of scans was read by two researchers without the help of AI. (Currently, the standard of care across Europe is to have two radiologists analyze a scan before diagnosing a patient with breast cancer.)
The study showed that the AI group detected cancer in 6 out of every 1,000 scans, while the radiologists detected cancer in 5 per 1,000 scans. In other words, AI found 20 percent more cancers than the highly-trained radiologists.
Scientists have been using MRI images (like the ones pictured here) to train artificial intelligence to detect cancers earlier and with more accuracy. Here, MIT's AI system, MIRAI, looks for patterns in a patient's mammograms to detect breast cancer earlier than ever before. news.mit.edu
But even though the AI was better able to pinpoint cancer on an image, it doesn’t mean radiologists will soon be out of a job. Dr. Laura Heacock, a breast radiologist at NYU, said in an interview with CNN that radiologists do much more than simply screening mammograms, and that even well-trained technology can make errors. “These tools work best when paired with highly-trained radiologists who make the final call on your mammogram. Think of it as a tool like a stethoscope for a cardiologist.”
AI is still an emerging technology, but more and more doctors are using them to detect different cancers. For example, researchers at MIT have developed a program called MIRAI, which looks at patterns in patient mammograms across a series of scans and uses an algorithm to model a patient's risk of developing breast cancer over time. The program was "trained" with more than 200,000 breast imaging scans from Massachusetts General Hospital and has been tested on over 100,000 women in different hospitals across the world. According to MIT, MIRAI "has been shown to be more accurate in predicting the risk for developing breast cancer in the short term (over a 3-year period) compared to traditional tools." It has also been able to detect breast cancer up to five years before a patient receives a diagnosis.
The challenges for cancer-detecting AI tools now is not just accuracy. AI tools are also being challenged to perform consistently well across different ages, races, and breast density profiles, particularly given the increased risks that different women face. For example, Black women are 42 percent more likely than white women to die from breast cancer, despite having nearly the same rates of breast cancer as white women. Recently, an FDA-approved AI device for screening breast cancer has come under fire for wrongly detecting cancer in Black patients significantly more often than white patients.
As AI technology improves, radiologists will be able to accurately scan a more diverse set of patients at a larger volume than ever before, potentially saving more lives than ever.