Scientists are making machines, wearable and implantable, to act as kidneys
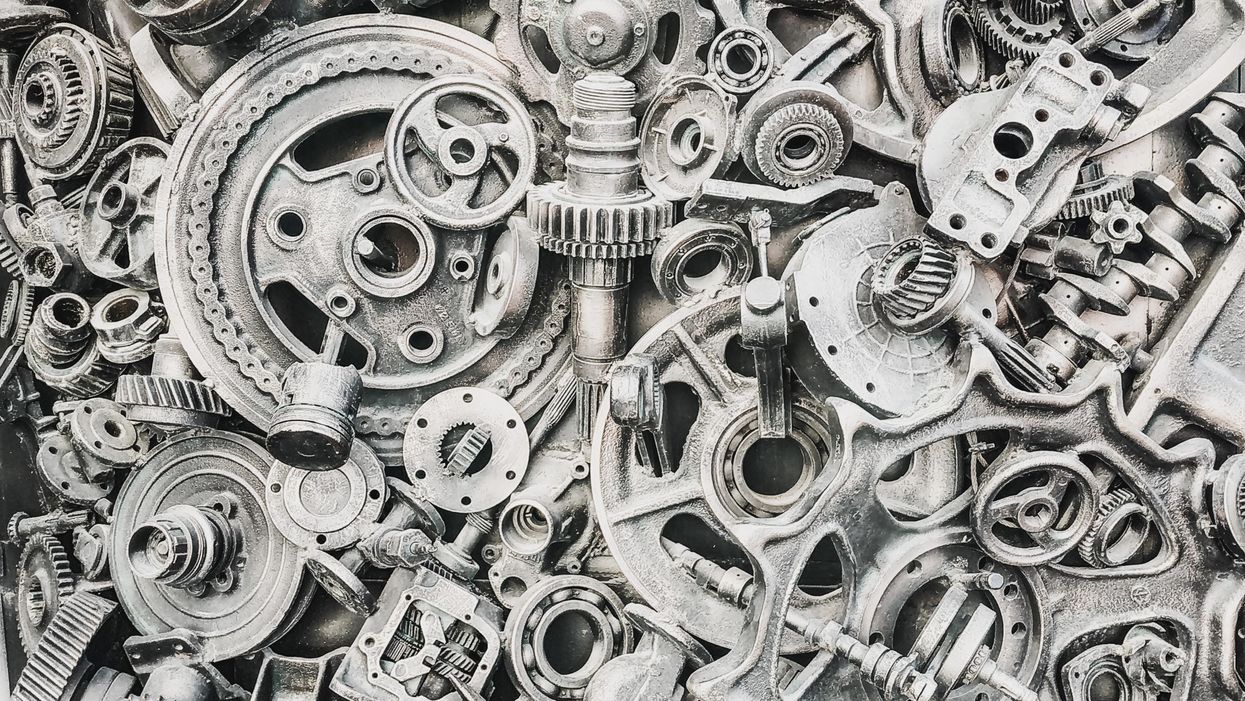
Recent advancements in engineering mean that the first preclinical trials for an artificial kidney could happen soon.
Like all those whose kidneys have failed, Scott Burton’s life revolves around dialysis. For nearly two decades, Burton has been hooked up (or, since 2020, has hooked himself up at home) to a dialysis machine that performs the job his kidneys normally would. The process is arduous, time-consuming, and expensive. Except for a brief window before his body rejected a kidney transplant, Burton has depended on machines to take the place of his kidneys since he was 12-years-old. His whole life, the 39-year-old says, revolves around dialysis.
“Whenever I try to plan anything, I also have to plan my dialysis,” says Burton says, who works as a freelance videographer and editor. “It’s a full-time job in itself.”
Many of those on dialysis are in line for a kidney transplant that would allow them to trade thrice-weekly dialysis and strict dietary limits for a lifetime of immunosuppressants. Burton’s previous transplant means that his body will likely reject another donated kidney unless it matches perfectly—something he’s not counting on. It’s why he’s enthusiastic about the development of artificial kidneys, small wearable or implantable devices that would do the job of a healthy kidney while giving users like Burton more flexibility for traveling, working, and more.
Still, the devices aren’t ready for testing in humans—yet. But recent advancements in engineering mean that the first preclinical trials for an artificial kidney could happen soon, according to Jonathan Himmelfarb, a nephrologist at the University of Washington.
“It would liberate people with kidney failure,” Himmelfarb says.
An engineering marvel
Compared to the heart or the brain, the kidney doesn’t get as much respect from the medical profession, but its job is far more complex. “It does hundreds of different things,” says UCLA’s Ira Kurtz.
Kurtz would know. He’s worked as a nephrologist for 37 years, devoting his career to helping those with kidney disease. While his colleagues in cardiology and endocrinology have seen major advances in the development of artificial hearts and insulin pumps, little has changed for patients on hemodialysis. The machines remain bulky and require large volumes of a liquid called dialysate to remove toxins from a patient’s blood, along with gallons of purified water. A kidney transplant is the next best thing to someone’s own, functioning organ, but with over 600,000 Americans on dialysis and only about 100,000 kidney transplants each year, most of those in kidney failure are stuck on dialysis.
Part of the lack of progress in artificial kidney design is the sheer complexity of the kidney’s job. Each of the 45 different cell types in the kidney do something different.
Part of the lack of progress in artificial kidney design is the sheer complexity of the kidney’s job. To build an artificial heart, Kurtz says, you basically need to engineer a pump. An artificial pancreas needs to balance blood sugar levels with insulin secretion. While neither of these tasks is simple, they are fairly straightforward. The kidney, on the other hand, does more than get rid of waste products like urea and other toxins. Each of the 45 different cell types in the kidney do something different, helping to regulate electrolytes like sodium, potassium, and phosphorous; maintaining blood pressure and water balance; guiding the body’s hormonal and inflammatory responses; and aiding in the formation of red blood cells.
There's been little progress for patients during Ira Kurtz's 37 years as a nephrologist. Artificial kidneys would change that.
UCLA
Dialysis primarily filters waste, and does so well enough to keep someone alive, but it isn’t a true artificial kidney because it doesn’t perform the kidney’s other jobs, according to Kurtz, such as sensing levels of toxins, wastes, and electrolytes in the blood. Due to the size and water requirements of existing dialysis machines, the equipment isn’t portable. Physicians write a prescription for a certain duration of dialysis and assess how well it’s working with semi-regular blood tests. The process of dialysis itself, however, is conducted blind. Doctors can’t tell how much dialysis a patient needs based on kidney values at the time of treatment, says Meera Harhay, a nephrologist at Drexel University in Philadelphia.
But it’s the impact of dialysis on their day-to-day lives that creates the most problems for patients. Only one-quarter of those on dialysis are able to remain employed (compared to 85% of similar-aged adults), and many report a low quality of life. Having more flexibility in life would make a major different to her patients, Harhay says.
“Almost half their week is taken up by the burden of their treatment. It really eats away at their freedom and their ability to do things that add value to their life,” she says.
Art imitates life
The challenge for artificial kidney designers was how to compress the kidney’s natural functions into a portable, wearable, or implantable device that wouldn’t need constant access to gallons of purified and sterilized water. The other universal challenge they faced was ensuring that any part of the artificial kidney that would come in contact with blood was kept germ-free to prevent infection.
As part of the 2021 KidneyX Prize, a partnership between the U.S. Department of Health and Human Services and the American Society of Nephrology, inventors were challenged to create prototypes for artificial kidneys. Himmelfarb’s team at the University of Washington’s Center for Dialysis Innovation won the prize by focusing on miniaturizing existing technologies to create a portable dialysis machine. The backpack sized AKTIV device (Ambulatory Kidney to Increase Vitality) will recycle dialysate in a closed loop system that removes urea from blood and uses light-based chemical reactions to convert the urea to nitrogen and carbon dioxide, which allows the dialysate to be recirculated.
Himmelfarb says that the AKTIV can be used when at home, work, or traveling, which will give users more flexibility and freedom. “If you had a 30-pound device that you could put in the overhead bins when traveling, you could go visit your grandkids,” he says.
Kurtz’s team at UCLA partnered with the U.S. Kidney Research Corporation and Arkansas University to develop a dialysate-free desktop device (about the size of a small printer) as the first phase of a progression that will he hopes will lead to something small and implantable. Part of the reason for the artificial kidney’s size, Kurtz says, is the number of functions his team are cramming into it. Not only will it filter urea from blood, but it will also use electricity to help regulate electrolyte levels in a process called electrodeionization. Kurtz emphasizes that these additional functions are what makes his design a true artificial kidney instead of just a small dialysis machine.
One version of an artificial kidney.
UCLA
“It doesn't have just a static function. It has a bank of sensors that measure chemicals in the blood and feeds that information back to the device,” Kurtz says.
Other startups are getting in on the game. Nephria Bio, a spinout from the South Korean-based EOFlow, is working to develop a wearable dialysis device, akin to an insulin pump, that uses miniature cartridges with nanomaterial filters to clean blood (Harhay is a scientific advisor to Nephria). Ian Welsford, Nephria’s co-founder and CTO, says that the device’s design means that it can also be used to treat acute kidney injuries in resource-limited settings. These potentials have garnered interest and investment in artificial kidneys from the U.S. Department of Defense.
For his part, Burton is most interested in an implantable device, as that would give him the most freedom. Even having a regular outpatient procedure to change batteries or filters would be a minor inconvenience to him.
“Being plugged into a machine, that’s not mimicking life,” he says.
This article was first published by Leaps.org on May 5, 2022.
The Women of RNA: Two Award-Winners Share Why They Spent Their Careers Studying DNA's Lesser-Known Cousin
When Lynne Maquat, who leads the Center for RNA Biology at the University of Rochester, became interested in the ribonucleic acid molecule in the 1970s, she was definitely in the minority. The same was true for Joan Steitz, now professor of molecular biophysics and biochemistry at Yale University, who began to study RNA a decade earlier in the 1960s.
"My first RNA experiment was a failure, because we didn't understand how things worked," Steitz recalls. In her first undergraduate experiment, she unwittingly used a lab preparation that destroyed the RNA. "Unknowingly, our preparation contained enzymes that degraded our RNA."
At the time, scientists pursuing genetic research tended to focus on DNA, or deoxyribonucleic acid — and for good reason. It was clear that the enigmatic double-helix ribbon held the answers to organisms' heredity, genetic traits, development, growth and aging. If scientists could decipher the secrets of DNA and understand how its genetic instructions translate into the body's functions in health and disease, they could develop treatments for all kinds of diseases. On the contrary, the prevailing dogma of the time viewed RNA as merely a helper that passively carried out DNA's genetic instructions for protein-making — so it received much less attention.
But Maquat and Steitz weren't interested in heredity. They studied biochemistry and biophysics, so they wanted to understand how RNA functioned on the molecular level — how it carried instructions, catalyzed reactions, and helped build protein bonds, among other things.
"I'm a mechanistic biochemist, so I like to know how things happen," Maquat says. "Once you understand the mechanism, you can think of how to solve problems." And so the quest to understand how RNA does its job became the focus of both women's careers.
"People can now appreciate why some of us studied RNA for such a long time."
Half a century later, in 2021, their RNA work has earned two prestigious recognitions only months from each other. In February, they received the Wolf Prize in Medicine, followed by the Warren Alpert Foundation Prize in May, awarded to scientists whose achievements led to prevention, cure or treatments of human diseases.
It was the development of the COVID-19 vaccines that made RNA a household name. Made by Moderna and Pfizer, the vaccines use the RNA molecule to deliver genetic instructions for making SARS-CoV-2's characteristic spike protein in our cells. The presence of this foreign-looking protein triggers the immune system to attack and remember the pathogen. As the vaccines reached the finish line, RNA took center stage, and it was Maquat's and Steitz's research that helped reveal how these molecular cogwheels drive many biological functions within cells.
If you think of a cell as a kingdom, the DNA plays the role of a queen. Like a monarch in a palace, DNA nestles inside the cell's nucleus issuing instructions needed for the cell to function. But no queen can successfully govern without her court, her messengers, and her soldiers, as well as other players that make her kingdom work. That's what RNAs do — they act as the DNA's vassals. They carry instructions for protein assembly, catalyze reactions and supervise many other processes to make sure the cellular kingdom performs as it should.
There are a myriad of these RNA vassals in our cells, and each type has its own specific task. There are messenger RNAs that deliver genetic instructions for protein synthesis from DNA to ribosomes, the cells' protein-making factories. There are ribosomal RNAs that help stitch together amino acids to make proteins. There are transfer RNAs that can bring amino acids to this protein synthesis machine, keeping it going. Then there are circular RNAs that act as sponges, absorbing proteins to help regulate the activity of genes. And that's only the tip of the iceberg when it comes to RNA diversity, researchers say.
"We know what the most abundant and important RNAs are doing," says Steitz. "But there are thousands of different ones, and we still don't have a full knowledge of them."
Critical to RNA's proper functioning is a process called splicing, in which a precursor mRNA is transformed into mature, fully-functional mRNA — a phenomenon that Steitz's work helped elucidate. The splicing process, which takes place in cellular assembly lines, involves removing extra RNA sequences and stringing the remaining RNA pieces together. Steitz found that tiny RNA particles called snRNPs are crucial to this process. They act as handy helpers, finding and removing errant genetic material from the mRNA molecules.
A dysfunctional RNA assembly line leads to diseases, including many cancers. For instance, Steitz found that people with Lupus — an autoimmune disorder — have antibodies that mistakenly attack the little snRNP helpers. She also discovered that when snRNPs don't do their job properly, they can cause what scientists call mis-splicing, producing defective mRNAs.
Fortunately, cells have a built-in quality-control process that can spot and correct these mistakes, which is what Maquat studied in her work. In 1981, she discovered a molecular quality-control system that spots and destroys such incorrectly assembled mRNA. With the cryptic name "nonsense-mediated mRNA decay" or NMD, this process is vital to the health and wellbeing of a cellular kingdom in humans — because splicing mistakes happen far more often than one would imagine.
"We estimate that about a third of our mRNA are mistakes," Maquat says. "And nonsense-mediated mRNA decay cleans up these mistakes." When this quality-control system malfunctions, defective mRNA forge faulty proteins, which mess up the cellular machinery and cause disease, including various forms of cancer.
Scientists' newfound appreciation of RNA opens door to many novel treatments.
Now that the first RNA-based shots were approved, the same principle can be used for create vaccines for other diseases, the two RNA researchers say. Moreover, the molecule has an even greater potential — it can serve as a therapeutic target for other disorders. For example, Spinraza, a groundbreaking drug approved in 2016 for spinal muscular atrophy, uses small snippets of synthetic genetic material that bind to the RNA, helping fix splicing errors. "People can now appreciate why some of us studied RNA for such a long time," says Maquat.
Steitz is thrilled that the entire field of RNA research is enjoying the limelight. "I'm delighted because the prize is more of a recognition of the field than just our work," she says. "This is a more general acknowledgment of how basic research can have a remarkable impact on human health."
Lina Zeldovich has written about science, medicine and technology for Popular Science, Smithsonian, National Geographic, Scientific American, Reader’s Digest, the New York Times and other major national and international publications. A Columbia J-School alumna, she has won several awards for her stories, including the ASJA Crisis Coverage Award for Covid reporting, and has been a contributing editor at Nautilus Magazine. In 2021, Zeldovich released her first book, The Other Dark Matter, published by the University of Chicago Press, about the science and business of turning waste into wealth and health. You can find her on http://linazeldovich.com/ and @linazeldovich.
In 2010, a 67-year-old former executive assistant for a Fortune 500 company was diagnosed with mild cognitive impairment. By 2014, her doctors confirmed she had Alzheimer's disease.
As her disease progressed, she continued to live independently but wasn't able to drive anymore. Today, she can manage most of her everyday tasks, but her two daughters are considering a live-in caregiver. Despite her condition, the woman may represent a beacon of hope for the approximately 44 million people worldwide living with Alzheimer's disease. The now 74-year-old is among a small cadre of Alzheimer's patients who have undergone an experimental ultrasound procedure aimed at slowing cognitive decline.
In November 2020, Elisa Konofagou, a professor of biomedical engineering and director of the Ultrasound and Elasticity Imaging Laboratory at Columbia University, and her team used ultrasound to noninvasively open the woman's blood-brain barrier. This barrier is a highly selective membrane of cells that prevents toxins and pathogens from entering the brain while allowing vital nutrients to pass through. This regulatory function means the blood-brain barrier filters out most drugs, making treating Alzheimer's and other brain diseases a challenge.
Ultrasound uses high-frequency sound waves to produce live images from the inside of the human body. But scientists think it could also be used to boost the effectiveness of Alzheimer's drugs, or potentially even improve brain function in dementia patients without the use of drugs.
The procedure, which involves a portable ultrasound system, is the culmination of 17 years of lab work. As part of a small clinical trial, scientists positioned a sensor transmitting ultrasound waves on top of the woman's head while she sat in a chair. The sensor sends ultrasound pulses throughout the target region. Meanwhile, investigators intravenously infused microbubbles into the woman to boost the effects of the ultrasound. Three days after the procedure, scientists scanned her brain so that they could measure the effects of the treatments. Five months later, they took more images of her brain to see if the effects of the treatment lasted.
Promising Signs
After the first brain scan, Konofagou and her team found that amyloid-beta, the protein that clumps together in the brains of Alzheimer's patients and disrupts cell function, had declined by 14%. At the woman's second scan, amyloid levels were still lower than before the experimental treatment, but only by 10% this time. Konofagou thinks repeat ultrasound treatments given early on in the development of Alzheimer's may have the best chance at keeping amyloid plaques at bay.
This reduction in amyloid appeared to halt the woman's cognitive decline, at least temporarily. Following the ultrasound treatment, the woman took a 30-point test used to measure cognitive impairment in Alzheimer's. Her score — 22, indicating mild cognitive impairment — remained the same as before the intervention. Konofagou says this was actually a good sign.
"Typically, every six months an Alzheimer's patient scores two to three points lower, so this is highly encouraging," she says.
Konofagou speculates that the results might have been even more impressive had they applied the ultrasound on a larger section of the brain at a higher frequency. The selected site was just 4 cubic centimeters. Current safety protocols set by the U.S. Food and Drug Administration stipulate that investigators conducting such trials only treat one brain region with the lowest pressure possible.
The Columbia trial is aided by microbubble technology. During the procedure, investigators infused tiny, gas-filled spheres into the woman's veins to enhance the ultrasound reflection of the sound waves.
The big promise of ultrasound is that it could eventually make drugs for Alzheimer's obsolete.
"Ultrasound with microbubbles wakes up immune cells that go on to discard amyloid-beta," Konofagou says. "In this way, we can recover the function of brain neurons, which are destroyed by Alzheimer's in a sort of domino effect." What's more, a drug delivered alongside ultrasound can penetrate the brain at a dose up to 10 times higher.
Costas Arvanitis, an assistant professor at Georgia Institute of Technology who studies ultrasonic biophysics and isn't involved in the Columbia trial, is excited about the research. "First, by applying ultrasound you can make larger drugs — picture an antibody — available to the brain," he says. Then, you can use ultrasound to improve the therapeutic index, or the ratio of the effectiveness of a drug versus the ratio of adverse effects. "Some drugs might be effective but because we have to provide them in high doses to see significant responses they tend to come with side effects. By improving locally the concentration of a drug, you open up the possibility to reduce the dose."
The Columbia trial will enroll just six patients and is designed to test the feasibility and safety of the approach, not its efficacy. Still, Arvantis is hopeful about the potential benefits of the technique. "The technology has already been demonstrated to be safe, its components are now tuned to the needs of this specific application, and it's safe to say it's only a matter of time before we are able to develop personalized treatments," he says.
Konofagou and her colleagues recently presented their findings at the 20th Annual International Symposium for Therapeutic Ultrasound and intend to publish them in a scientific journal later this year. They plan to recruit more participants for larger trials, which will determine how effective the therapy is at improving memory and brain function in Alzheimer's patients. They're also in talks with pharmaceutical companies about ways to use their therapeutic approach to improve current drugs or even "create new drugs," says Konofagou.
A New Treatment Approach
On June 7, the FDA approved the first Alzheimer's disease drug in nearly two decades. Aducanumab, a drug developed by Biogen, is an antibody designed to target and reduce amyloid plaques. The drug has already sparked immense enthusiasm — and controversy. Proponents say the drug is a much-needed start in the fight against the disease, but others argue that the drug doesn't substantially improve cognition. They say the approval could open the door to the FDA greenlighting more Alzheimer's drugs that don't have a clear benefit, giving false hope to both patients and their families.
Konofagou's ultrasound approach could potentially boost the effects of drugs like aducanumab. "Our technique can be seamlessly combined with aducanumab in early Alzheimer's, where it has shown the most promise, to further enhance both its amyloid load reduction and further reduce cognitive deficits while using exactly the same drug regimen otherwise," she says. For the Columbia team, the goal is to use ultrasound to maximize the effects of aducanumab, as they've done with other drugs in animal studies.
But Konofagou's approach could transcend drug controversies, and even drugs altogether. The big promise of ultrasound is that it could eventually make drugs for Alzheimer's obsolete.
"There are already indications that the immune system is alerted each time ultrasound is exerted on the brain or when the brain barrier is being penetrated and gets activated, which on its own may have sufficient therapeutic effects," says Konofagou. Her team is now working with psychiatrists in hopes of using brain stimulation to treat patients with depression.
The potential to modulate the brain without drugs is huge and untapped, says Kim Butts Pauly, a professor of radiology, electrical engineering and bioengineering at Stanford University, who's not involved in the Columbia study. But she admits that scientists don't know how to fully control ultrasound in the brain yet. "We're only at the starting point of getting the tools to understand and harness how ultrasound microbubbles stimulate an immune response in the brain."
Meanwhile, the 74-year-old woman who received the ultrasound treatment last year, goes on about her life, having "both good days and bad days," her youngest daughter says. COVID-19's isolation took a toll on her, but both she and her daughters remain grateful for the opportunity to participate in the ultrasound trial.
"My mother wants to help, if not for herself, then for those who will follow her," the daughter says. She hopes her mother will be able to join the next phase of the trial, which will involve a drug in conjunction with the ultrasound treatment. "This may be the combination where the magic will happen," her daughter says.