Scientists are making machines, wearable and implantable, to act as kidneys
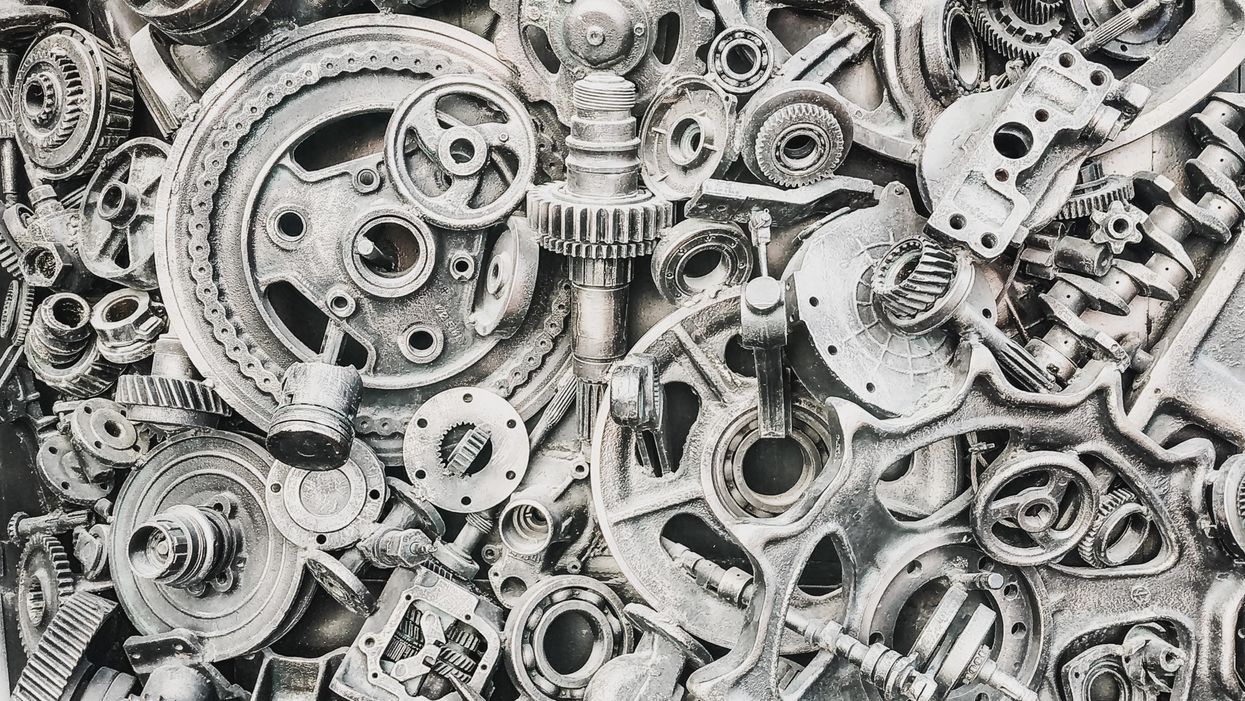
Recent advancements in engineering mean that the first preclinical trials for an artificial kidney could happen as soon as 18 months from now
Like all those whose kidneys have failed, Scott Burton’s life revolves around dialysis. For nearly two decades, Burton has been hooked up (or, since 2020, has hooked himself up at home) to a dialysis machine that performs the job his kidneys normally would. The process is arduous, time-consuming, and expensive. Except for a brief window before his body rejected a kidney transplant, Burton has depended on machines to take the place of his kidneys since he was 12-years-old. His whole life, the 39-year-old says, revolves around dialysis.
“Whenever I try to plan anything, I also have to plan my dialysis,” says Burton says, who works as a freelance videographer and editor. “It’s a full-time job in itself.”
Many of those on dialysis are in line for a kidney transplant that would allow them to trade thrice-weekly dialysis and strict dietary limits for a lifetime of immunosuppressants. Burton’s previous transplant means that his body will likely reject another donated kidney unless it matches perfectly—something he’s not counting on. It’s why he’s enthusiastic about the development of artificial kidneys, small wearable or implantable devices that would do the job of a healthy kidney while giving users like Burton more flexibility for traveling, working, and more.
Still, the devices aren’t ready for testing in humans—yet. But recent advancements in engineering mean that the first preclinical trials for an artificial kidney could happen as soon as 18 months from now, according to Jonathan Himmelfarb, a nephrologist at the University of Washington.
“It would liberate people with kidney failure,” Himmelfarb says.
An engineering marvel
Compared to the heart or the brain, the kidney doesn’t get as much respect from the medical profession, but its job is far more complex. “It does hundreds of different things,” says UCLA’s Ira Kurtz.
Kurtz would know. He’s worked as a nephrologist for 37 years, devoting his career to helping those with kidney disease. While his colleagues in cardiology and endocrinology have seen major advances in the development of artificial hearts and insulin pumps, little has changed for patients on hemodialysis. The machines remain bulky and require large volumes of a liquid called dialysate to remove toxins from a patient’s blood, along with gallons of purified water. A kidney transplant is the next best thing to someone’s own, functioning organ, but with over 600,000 Americans on dialysis and only about 100,000 kidney transplants each year, most of those in kidney failure are stuck on dialysis.
Part of the lack of progress in artificial kidney design is the sheer complexity of the kidney’s job. Each of the 45 different cell types in the kidney do something different.
Part of the lack of progress in artificial kidney design is the sheer complexity of the kidney’s job. To build an artificial heart, Kurtz says, you basically need to engineer a pump. An artificial pancreas needs to balance blood sugar levels with insulin secretion. While neither of these tasks is simple, they are fairly straightforward. The kidney, on the other hand, does more than get rid of waste products like urea and other toxins. Each of the 45 different cell types in the kidney do something different, helping to regulate electrolytes like sodium, potassium, and phosphorous; maintaining blood pressure and water balance; guiding the body’s hormonal and inflammatory responses; and aiding in the formation of red blood cells.
There's been little progress for patients during Ira Kurtz's 37 years as a nephrologist. Artificial kidneys would change that.
UCLA
Dialysis primarily filters waste, and does so well enough to keep someone alive, but it isn’t a true artificial kidney because it doesn’t perform the kidney’s other jobs, according to Kurtz, such as sensing levels of toxins, wastes, and electrolytes in the blood. Due to the size and water requirements of existing dialysis machines, the equipment isn’t portable. Physicians write a prescription for a certain duration of dialysis and assess how well it’s working with semi-regular blood tests. The process of dialysis itself, however, is conducted blind. Doctors can’t tell how much dialysis a patient needs based on kidney values at the time of treatment, says Meera Harhay, a nephrologist at Drexel University in Philadelphia.
But it’s the impact of dialysis on their day-to-day lives that creates the most problems for patients. Only one-quarter of those on dialysis are able to remain employed (compared to 85% of similar-aged adults), and many report a low quality of life. Having more flexibility in life would make a major different to her patients, Harhay says.
“Almost half their week is taken up by the burden of their treatment. It really eats away at their freedom and their ability to do things that add value to their life,” she says.
Art imitates life
The challenge for artificial kidney designers was how to compress the kidney’s natural functions into a portable, wearable, or implantable device that wouldn’t need constant access to gallons of purified and sterilized water. The other universal challenge they faced was ensuring that any part of the artificial kidney that would come in contact with blood was kept germ-free to prevent infection.
As part of last year’s KidneyX Prize, a partnership between the U.S. Department of Health and Human Services and the American Society of Nephrology, inventors were challenged to create prototypes for artificial kidneys. Himmelfarb’s team at the University of Washington’s Center for Dialysis Innovation won the prize by focusing on miniaturizing existing technologies to create a portable dialysis machine. The backpack sized AKTIV device (Ambulatory Kidney to Increase Vitality) will recycle dialysate in a closed loop system that removes urea from blood and uses light-based chemical reactions to convert the urea to nitrogen and carbon dioxide, which allows the dialysate to be recirculated.
Himmelfarb says that the AKTIV can be used when at home, work, or traveling, which will give users more flexibility and freedom. “If you had a 30-pound device that you could put in the overhead bins when traveling, you could go visit your grandkids,” he says.
Kurtz’s team at UCLA partnered with the U.S. Kidney Research Corporation and Arkansas University to develop a dialysate-free desktop device (about the size of a small printer) as the first phase of a progression that will he hopes will lead to something small and implantable. Part of the reason for the artificial kidney’s size, Kurtz says, is the number of functions his team are cramming into it. Not only will it filter urea from blood, but it will also use electricity to help regulate electrolyte levels in a process called electrodeionization. Kurtz emphasizes that these additional functions are what makes his design a true artificial kidney instead of just a small dialysis machine.
One version of an artificial kidney.
UCLA
“It doesn't have just a static function. It has a bank of sensors that measure chemicals in the blood and feeds that information back to the device,” Kurtz says.
Other startups are getting in on the game. Nephria Bio, a spinout from the South Korean-based EOFlow, is working to develop a wearable dialysis device, akin to an insulin pump, that uses miniature cartridges with nanomaterial filters to clean blood (Harhay is a scientific advisor to Nephria). Ian Welsford, Nephria’s co-founder and CTO, says that the device’s design means that it can also be used to treat acute kidney injuries in resource-limited settings. These potentials have garnered interest and investment in artificial kidneys from the U.S. Department of Defense.
For his part, Burton is most interested in an implantable device, as that would give him the most freedom. Even having a regular outpatient procedure to change batteries or filters would be a minor inconvenience to him.
“Being plugged into a machine, that’s not mimicking life,” he says.
How Genetic Testing and Targeted Treatments Are Helping More Cancer Patients Survive
Scientists are studying cancer genomes to more precisely diagnose their patients' diseases - offering hope for targeted drug treatments.
Late in 2018, Chris Reiner found himself “chasing a persistent cough” to figure out a cause. He talked to doctors; he endured various tests, including an X-ray. Initially, his physician suspected bronchitis. After several months, he still felt no improvement. In May 2019, his general practitioner recommended that Reiner, a business development specialist for a Seattle-based software company, schedule a CAT scan.
Reiner knew immediately that his doctor asking him to visit his office to discuss the results wasn’t a good sign. The longtime resident of Newburyport, MA, remembers dreading “that conversation that people who learn they have cancer have.”
“The doctor handed me something to look at, and the only thing I remember after that was everything went blank all around me,” Reiner, 50, reveals. “It was the magnitude of what he was telling me, that I had a malignant mass in my lung.”
Next, he recalls, he felt ushered into “the jaws of the medical system very quickly.” He spent a couple of days meeting with a team of doctors at Beth Israel Deaconess Medical Center in nearby Boston. One of them was from a medical field he hadn’t even known existed, a pulmonary interventionist, who would perform a biopsy on the mass in his lung.
“Knowing there was a medicine for my particular type of cancer was like a weight lifted off my shoulders."
A week later he and his wife Allison returned to meet with the oncologist, radiologist, pulmonary interventionist – his medical team. They confirmed his initial diagnosis: Stage 4 metastatic lung cancer that had spread to several parts of his body. “We just sat there, stunned,” he says. “I felt like I was getting hit by a wrecking ball over and over.”
An onslaught of medical terminology about what they had identified flowed over the shocked couple, but then the medical team switched gears, he recalls. They offered hope. “They told me, ‘Hey, you’re not a smoker, so that’s good,’” Reiner says. “‘There’s a good chance that what’s driving this disease for you is actually a genetic mutation, and we have ways to understand more about what that could be through some simple testing.’”
They told him about Foundation Medicine, a company launched in neighboring Cambridge, MA, in 2009 that develops, manufactures, and sells genomic profiling assays. These are tests that, according to the company’s website, “can analyze a broad panel of genes to detect the four main classes of genomic alterations known to drive cancer growth.” With these insights, certain patients can be matched with therapies targeted specifically for the genetic driver(s) of their cancer. The company maintains one of the largest cancer genomic databases in the world, with more than 500,000 patient samples profiled, and they have more than 65 biopharma partners.
According to Foundation Medicine, they are the only company that has FDA-approved tests for both tissue- and blood-based comprehensive genomic profiling tests. One other company has an FDA-approved biopsy test, and several other companies offer tissue-based genomic profiling. Additionally, several major cancer centers like Memorial Sloan Kettering in New York and Anderson Cancer Center in Texas have their own such testing platforms.
Currently, genomic profiling is more accessible for patients with advanced cancer, due to broader insurance coverage in later stages of disease.
“Right now, the vast majority of patients either have cancers for which we don’t have treatments or they have genetic alterations that are not known,” says Jorge Garcia, MD, Division Chief, Solid Tumor Oncology, UH Cleveland Medical Center, which has its own CGP testing platform. “However, a significant proportion of patients with advanced cancer have alterations that we can tap for therapeutic purposes.”
Foundation Medicine estimates that in 2017, just over 5 percent of advanced solid cancer patients in the U.S. received CGP testing. In 2021, they estimate that number is between 25 to 30 percent of advanced solid cancer patients in the U.S., which doesn’t include patients who are tested with small (less than 50 genes) panels. Their panel tests for more than 300 cancer-related genes.
“The good news is the platforms we are developing are better and more comprehensive, and they’re going to continue to be larger data sets,” Dr. Garcia adds.
In Reiner’s case, his team ordered comprehensive genetic profiling on both his tissue and blood, from Foundation Medicine.
At this point, Reiner still wasn’t sure what genetic mutations were or how they factored into cancer or what comprehensive genomic profiling entailed. That day, though, his team ushered the Reiners into the world of precision oncology that placed him on much more sure footing to learn about and fight the specific lung cancer that had been troubling him for more than a year.
What genetic alterations were driving his cancer? Foundation Medicine’s tests were about to find out.
At the core of these tests is next generation sequencing, a DNA sequencing technology. Since 2009, this has revolutionized genomic research, according to the National Center for Biotechnology Information, because it allows an entire human genome to be sequenced within one day. Cancer genomics posits that cancer is caused by mutations and is a disease of the genome. Now, cancer genomes can be systemically studied in their entirety. For cancer patients such as Reiner, NGS can provide a more precise diagnosis and classification of the disease, more accurate prognosis, and potentially the identification of targeted drug treatments. Ultimately, the technology can provide the basis of personalized cancer management.
The detailed reports supply patients and their oncologists with extensive information about the patient’s genomic profile and potential treatment options that they can discuss together. Reiner trusted his doctors that this approach was worth the two- or three-week wait to receive the Foundation Medicine report and the specifically targeted treatment, rather than immediately jump into a round of chemotherapy. He is especially grateful now, he says, because the report delivered a great deal of relief from his previously exhausting and growing anxiety about having cancer.
Reiner and his team learned his lung cancer contained the epidermal growth factor receptor (EGFR) mutation. That biomarker enabled his oncologist to prescribe Tagrisso (osimertinib), a medication developed to directly target that genetic mutation.
“Knowing there was a medicine for my particular type of cancer was like a weight lifted off my shoulders,” he says. “It only took a week or two before my cough finally started subsiding. This pill goes right after the particular piece of genetic material in the tumor that’s causing its growth.”
Dr. Jerry Mitchell, director field medical oncology, Foundation Medicine, in Columbus, Ohio, explains that genomic profiling is generating substantial impacts today. “This is a technology that is the standard of care across many advanced malignancies that takes patients from chemotherapy-only options to very targeted options or immunotherapy options,” he says. “You can also look at complex biomarkers, and these are not specific genetic changes but different genes across the tumor to get a biomarker.”
According to Dr. Mitchell, Foundation Medicine’s technology can test more than 324 different cancer-related genes in a single test. Thus, a growing number of patients are benefitting from comprehensive genetic profiling, due to the rapidly growing number of targeted therapies. While not all of the cancers are treatable yet, the company uses that information to partner with researchers to find new potential therapies for patient groups that may have rare mutations.
Since his tumor’s diagnosis, Reiner has undergone chemotherapy and a couple surgeries to treat the metastatic cancer in other parts of his body, but the drug Tagrisso has significantly reduced his lung tumor. Now, having learned so much during the past couple of years, he is grateful for precision oncology. He still reflects on the probability that, had the Tagrisso pill not been available in May 2019, he might have only survived for another six months or a year.
“Comprehensive Genomic Profiling is not some future state, but in both the U.S. and Europe, it is a very standard, accepted, and recommended first step to knowing how to treat your cancer,” says Dr. Mitchell, adding that he feels fortunate to be an oncologist in this era. “However, we know there are still people not getting this recommended testing, so we still have opportunities to find many more patients and impact them by knowing the molecular profile of their cancer.”
The Cellular Secrets of “Young Blood” Are Starting to Be Unlocked
Fabrisia Ambrosio (center) surrounded by her lab staff.
The quest for an elixir to restore youthful health and vigor is common to most cultures and has prompted much scientific research. About a decade ago, Stanford scientists stitched together the blood circulatory systems of old and young mice in a practice called parabiosis. It seemed to rejuvenate the aged animals and spawned vampirish urban legends of Hollywood luminaries and tech billionaires paying big bucks for healthy young blood to put into their own aging arteries in the hope of reversing or at least forestalling the aging process.
It was “kind of creepy” and also inspiring to Fabrisia Ambrosio, then thousands of miles away and near the start of her own research career into the processes of aging. Her lab is at the University of Pittsburgh but on this cold January morning I am speaking with her via Zoom as she visits with family near her native Sao Paulo, Brazil. A gleaming white high rise condo and a lush tropical jungle split the view behind her, and the summer beach is just a few blocks away.
Ambrosio possesses the joy of a kid on Christmas morning who can't wait to see what’s inside the wrapping. “I’ve always had a love for research, my father was a physicist," she says, but interest in the human body pulled her toward biology as her education progressed in the U.S. and Canada.
Back in Pittsburgh, her lab first extended the work of others in aging by using the simpler process of injecting young blood into the tail vein of old mice and found that the skeletal muscles of the animals “displayed an enhanced capacity to regenerate.” But what was causing this improvement?
When Ambrosio injected old mice with young blood depleted of EVs, the regenerative effect practically disappeared.
The next step was to remove the extracellular vesicles (EVs) from blood. EVs are small particles of cells composed of a membrane and often a cargo inside that lipid envelope. Initially many scientists thought that EVs were simply taking out the garbage that cells no longer needed, but they would learn that one cell's trash could be another cell's treasure.
Metabolites, mRNA, and myriad other signaling molecules inside the EV can function as a complex network by which cells communicate with others both near and far. These cargoes can up and down-regulate gene expression, affecting cell activity and potentially the entire body. EVs are present in humans, the bacteria that live in and on us, even in plants; they likely communicate across all forms of life.
Being inside the EV membrane protects cargo from enzymes and other factors in the blood that can degrade it, says Kenneth Witwer, a researcher at Johns Hopkins University and program chair of the International Society for Extracellular Vesicles. The receptors on the surface of the EV provide clues to the type of cell from which it originated and the cell receptors to which it might later bind and affect.
When Ambrosio injected old mice with young blood depleted of EVs, the regenerative effect practically disappeared; purified EVs alone were enough to do the job. The team also looked at muscle cell gene expression after injections of saline, young blood, and EV-depleted young blood and found significant differences. She believes this means that the major effect of enhanced regenerative capacity was coming from the EVs, though free floating proteins within the blood may also contribute something to the effect.
One such protein, called klotho, is of great interest to researchers studying aging. The name was borrowed from the Fates of Greek mythology, which consists of three sisters; Klotho spins the thread of life that her sisters measure and cut. Ambrosio had earlier shown that supplementing klotho could enhance regenerative capacity in old animals. But as with most proteins, klotho is fragile, rapidly degrading in body fluids, or when frozen and thawed. She suspected that klotho could survive better as cargo enclosed within the membrane of an EV and shielded from degradation.
So she went looking for klotho inside the EVs they had isolated. Advanced imaging technology revealed that young EVs contained abundant levels of klotho mRNAs, but the number of those proteins was much lower in EVs from old mice. Ambrosio wrote in her most recent paper, published in December in Nature Aging. She also found that the stressors associated with aging reduced the communications capacity of EVs in muscle tissue and that could be only partially restored with young blood.
Researchers still don't understand how klotho functions at the cellular level, but they may not need to know that. Perhaps learning how to increase its production, or using synthetic biology to generate more copies of klotho mRNA, or adding cell receptors to better direct EVs to specific aging tissue will be sufficient to reap the anti-aging benefits.
“Very, very preliminary data from our lab has demonstrated that exercise may be altering klotho transcripts within aged extracellular vesicles" for the better Ambrosio teases. But we already know that exercise is good for us; understanding the cellular mechanism behind that isn't likely to provide additional motivation to get up off the couch. Many of us want a prescription, a pill that is easy to take, to slow our aging.
Ambrosio hopes that others will build upon the basic research from her lab, and that pharmaceutical companies will be able to translate and develop it into products that can pass through FDA review and help ameliorate the diseases of aging.