Where Are the Lab-Grown Replacement Organs?
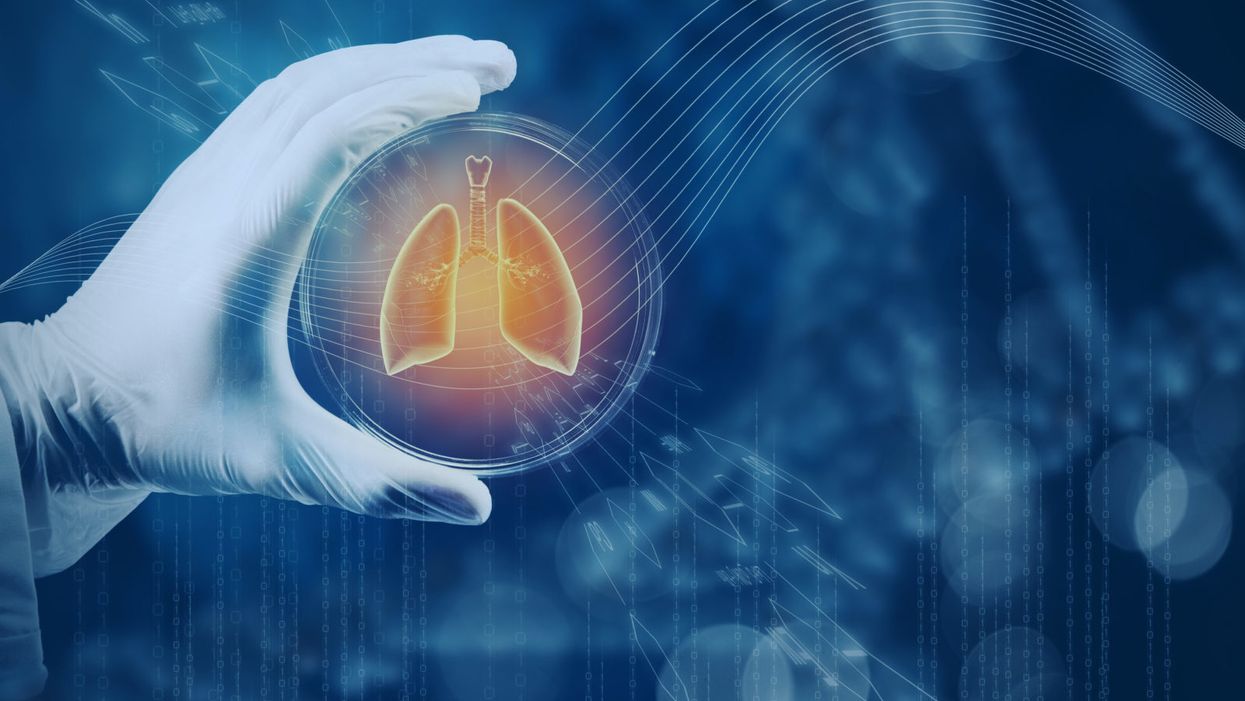
A futuristic rendering of a scientist holding a pair of lungs in a glass dish.
The headline blared from newspapers all the way back in 2006: "First Lab-Grown Organs Implanted in Humans!" A team from Wake Forest University had biopsied cells from the bladders of patients with spina bifida and used them to create brand new full-size bladders, which they then implanted. Although the bladders had to be emptied via catheter, they were still functioning a few years after implantation, and the public grew confident that doctors had climbed an intermediary step on the way to the medicine of science fiction. Ten years later, though, more than 20 people a day are still dying while waiting for an organ transplant, which leads to a simple question: Where are our fake organs?
"We can make small organs and tissues but we can't make larger ones."
Not coming anytime soon, unfortunately. The company that was created to transition Wake Forest's bladders to the market failed. And while there are a few simple bioengineered skins and cartilages already on the market, they are hardly identical to the real thing. Something like a liver could take another 20 to 25 years, says Shay Soker, professor at Wake Forest's Institute for Regenerative Medicine. "The first barrier is the technology: We can make small organs and tissues but we can't make larger ones," he says. "Also there are several cell types or functions that you can reliably make from stem cells, but not all of them, so the technology of stem cells has to catch up with what the body can do." Finally, he says, you have support the new organ inside the body, providing it with a circulatory and nervous system and integrating it with the immune system.
While these are all challenging problems, circulation appears to be the most intractable. "Tissue's not able to survive if the cells don't have oxygen, and the bigger it gets, the more complex vasculature you need to keep that alive," says Chiara Ghezzi, research professor in the Tufts University Department of Biomedical Engineering. "Vasculature is highly organized in the body. It has a hierarchical structure, with different branches that have different roles depending on where they are." So far, she says, researchers have had trouble scaling up from capillaries to larger vessels that could be grafted onto blood vessels in a patient's body.
"The FDA is still getting its hands and minds around the field of tissue engineering."
Last, but hardly least, is the question of FDA approval. Lab-grown organs are neither drugs nor medical devices, and the agency is not set up to quickly or easily approve new technologies that don't fit into current categories. "The FDA is still getting its hands and minds around the field of tissue engineering," says Soker. "They were not used to that… so it requires the regulatory and financial federal agencies to really help and support these initiatives."
A pencil eraser-size model of the human brain is now being used for drug development and research.
If all of this sounds discouraging, it's worth mentioning some of the incredible progress the field has made since the first strides toward lab-grown organs began nearly 30 years ago: Though full-size replacement organs are still decades away, many labs have diverted their resources into what they consider an intermediate step, developing miniature organs and systems that can be used for drug development and research. This platform will yield more relevant results (Imagine! Testing cardiovascular drugs on an actual human heart!) and require the deaths of far fewer animals. And it's already here: Two years ago, scientists at Ohio State University developed a pencil eraser-size model of the human brain they intend to use for this exact purpose.
Perhaps the most exciting line of research these days is one that at first doesn't seem to have anything to do with bioengineered organs at all. Along with his colleagues, Chandan Sen, Director of the Center for Regenerative Medicine and Cell-based Therapies at Ohio State University, has developed a nanoscale chip that can turn any cell in the body into any other kind of cell—reverting fully differentiated adult cells into, essentially, stem cells, which can then grow into any tissue you want. Sen has used his chip to reprogram skin cells in the bodies of mice into neurons to help them recover from strokes, and blood vessels to save severe leg injuries. "There's this concept of a bioreactor, where you convince an organ to grow outside the body. They're getting more and more sophisticated over time. But to my mind it will never match the sophistication or complexity of the human body," Sen says. "I believe that in order to have an organ that behaves the way you want it to in the live body, you must use the body itself as a bioreactor, not a bunch of electronic gadgetry." There you have it, the next step in artificial organ manufacture is as crazy as it is intuitive: Grow it back where it was in the first place.
Fast for Longevity, with Less Hunger, with Dr. Valter Longo
Valter Longo, a biogerontologist at USC, and centenarian Rocco Longo (no relation) appear together in Italy in 2021. The elder Longo is from a part of Italy where people have fasted regularly and are enjoying long lifespans.
You’ve probably heard about intermittent fasting, where you don’t eat for about 16 hours each day and limit the window where you’re taking in food to the remaining eight hours.
But there’s another type of fasting, called a fasting-mimicking diet, with studies pointing to important benefits. For today’s podcast episode, I chatted with Dr. Valter Longo, a biogerontologist at the University of Southern California, about all kinds of fasting, and particularly the fasting-mimicking diet, which minimizes hunger as much as possible. Going without food for a period of time is an example of good stress: challenges that work at the cellular level to boost health and longevity.
Listen on Apple | Listen on Spotify | Listen on Stitcher | Listen on Amazon | Listen on Google
If you’ve ever spent more than a few minutes looking into fasting, you’ve almost certainly come upon Dr. Longo's name. He is the author of the bestselling book, The Longevity Diet, and the best known researcher of fasting-mimicking diets.
With intermittent fasting, your body might begin to switch up its fuel type. It's usually running on carbs you get from food, which gets turned into glucose, but without food, your liver starts making something called ketones, which are molecules that may benefit the body in a number of ways.
With the fasting-mimicking diet, you go for several days eating only types of food that, in a way, keep themselves secret from your body. So at the level of your cells, the body still thinks that it’s fasting. This is the best of both worlds – you’re not completely starving because you do take in some food, and you’re getting the boosts to health that come with letting a fast run longer than intermittent fasting. In this episode, Dr. Longo talks about the growing number of studies showing why this could be very advantageous for health, as long as you undertake the diet no more than a few times per year.
Dr. Longo is the director of the Longevity Institute at USC’s Leonard Davis School of Gerontology, and the director of the Longevity and Cancer program at the IFOM Institute of Molecular Oncology in Milan. In addition, he's the founder and president of the Create Cures Foundation in L.A., which focuses on nutrition for the prevention and treatment of major chronic illnesses. In 2016, he received the Glenn Award for Research on Aging for the discovery of genes and dietary interventions that regulate aging and prevent diseases. Dr. Longo received his PhD in biochemistry from UCLA and completed his postdoc in the neurobiology of aging and Alzheimer’s at USC.
Show links:
Create Cures Foundation, founded by Dr. Longo: www.createcures.org
Dr. Longo's Facebook: https://www.facebook.com/profvalterlongo/
Dr. Longo's Instagram: https://www.instagram.com/prof_valterlongo/
Dr. Longo's book: The Longevity Diet
The USC Longevity Institute: https://gero.usc.edu/longevity-institute/
Dr. Longo's research on nutrition, longevity and disease: https://pubmed.ncbi.nlm.nih.gov/35487190/
Dr. Longo's research on fasting mimicking diet and cancer: https://pubmed.ncbi.nlm.nih.gov/34707136/
Full list of Dr. Longo's studies: https://pubmed.ncbi.nlm.nih.gov/?term=Longo%2C+Valter%5BAuthor%5D&sort=date
Research on MCT oil and Alzheimer's: https://alz-journals.onlinelibrary.wiley.com/doi/f...
Keto Mojo device for measuring ketones
Silkworms with spider DNA spin silk stronger than Kevlar
Silkworm silk is fragile, which limits its uses, but a few extra genes can help.
Story by Freethink
The study and copying of nature’s models, systems, or elements to address complex human challenges is known as “biomimetics.” Five hundred years ago, an elderly Italian polymath spent months looking at the soaring flight of birds. The result was Leonardo da Vinci’s biomimetic Codex on the Flight of Birds, one of the foundational texts in the science of aerodynamics. It’s the science that elevated the Wright Brothers and has yet to peak.
Today, biomimetics is everywhere. Shark-inspired swimming trunks, gecko-inspired adhesives, and lotus-inspired water-repellents are all taken from observing the natural world. After millions of years of evolution, nature has quite a few tricks up its sleeve. They are tricks we can learn from. And now, thanks to some spider DNA and clever genetic engineering, we have another one to add to the list.
The elusive spider silk
We’ve known for a long time that spider silk is remarkable, in ways that synthetic fibers can’t emulate. Nylon is incredibly strong (it can support a lot of force), and Kevlar is incredibly tough (it can absorb a lot of force). But neither is both strong and tough. In all artificial polymeric fibers, strength and toughness are mutually exclusive, and so we pick the material best for the job and make do.
Spider silk, a natural polymeric fiber, breaks this rule. It is somehow both strong and tough. No surprise, then, that spider silk is a source of much study.The problem, though, is that spiders are incredibly hard to cultivate — let alone farm. If you put them together, they will attack and kill each other until only one or a few survive. If you put 100 spiders in an enclosed space, they will go about an aggressive, arachnocidal Hunger Games. You need to give each its own space and boundaries, and a spider hotel is hard and costly. Silkworms, on the other hand, are peaceful and productive. They’ll hang around all day to make the silk that has been used in textiles for centuries. But silkworm silk is fragile. It has very limited use.
The elusive – and lucrative – trick, then, would be to genetically engineer a silkworm to produce spider-quality silk. So far, efforts have been fruitless. That is, until now.
We can have silkworms creating silk six times as tough as Kevlar and ten times as strong as nylon.
Spider-silkworms
Junpeng Mi and his colleagues working at Donghua University, China, used CRISPR gene-editing technology to recode the silk-creating properties of a silkworm. First, they took genes from Araneus ventricosus, an East Asian orb-weaving spider known for its strong silk. Then they placed these complex genes – genes that involve more than 100 amino acids – into silkworm egg cells. (This description fails to capture how time-consuming, technical, and laborious this was; it’s a procedure that requires hundreds of thousands of microinjections.)
This had all been done before, and this had failed before. Where Mi and his team succeeded was using a concept called “localization.” Localization involves narrowing in on a very specific location in a genome. For this experiment, the team from Donghua University developed a “minimal basic structure model” of silkworm silk, which guided the genetic modifications. They wanted to make sure they had the exactly right transgenic spider silk proteins. Mi said that combining localization with this basic structure model “represents a significant departure from previous research.” And, judging only from the results, he might be right. Their “fibers exhibited impressive tensile strength (1,299 MPa) and toughness (319 MJ/m3), surpassing Kevlar’s toughness 6-fold.”
A world of super-materials
Mi’s research represents the bursting of a barrier. It opens up hugely important avenues for future biomimetic materials. As Mi puts it, “This groundbreaking achievement effectively resolves the scientific, technical, and engineering challenges that have hindered the commercialization of spider silk, positioning it as a viable alternative to commercially synthesized fibers like nylon and contributing to the advancement of ecological civilization.”
Around 60 percent of our clothing is made from synthetic fibers like nylon, polyester, and acrylic. These plastics are useful, but often bad for the environment. They shed into our waterways and sometimes damage wildlife. The production of these fibers is a source of greenhouse gas emissions. Now, we have a “sustainable, eco-friendly high-strength and ultra-tough alternative.” We can have silkworms creating silk six times as tough as Kevlar and ten times as strong as nylon.
We shouldn’t get carried away. This isn’t going to transform the textiles industry overnight. Gene-edited silkworms are still only going to produce a comparatively small amount of silk – even if farmed in the millions. But, as Mi himself concedes, this is only the beginning. If Mi’s localization and structure-model techniques are as remarkable as they seem, then this opens up the door to a great many supermaterials.
Nature continues to inspire. We had the bird, the gecko, and the shark. Now we have the spider-silkworm. What new secrets will we unravel in the future? And in what exciting ways will it change the world?
This article originally appeared on Freethink, home of the brightest minds and biggest ideas of all time.