The Inside Story of Two Young Scientists Who Helped Make Moderna's Covid Vaccine Possible
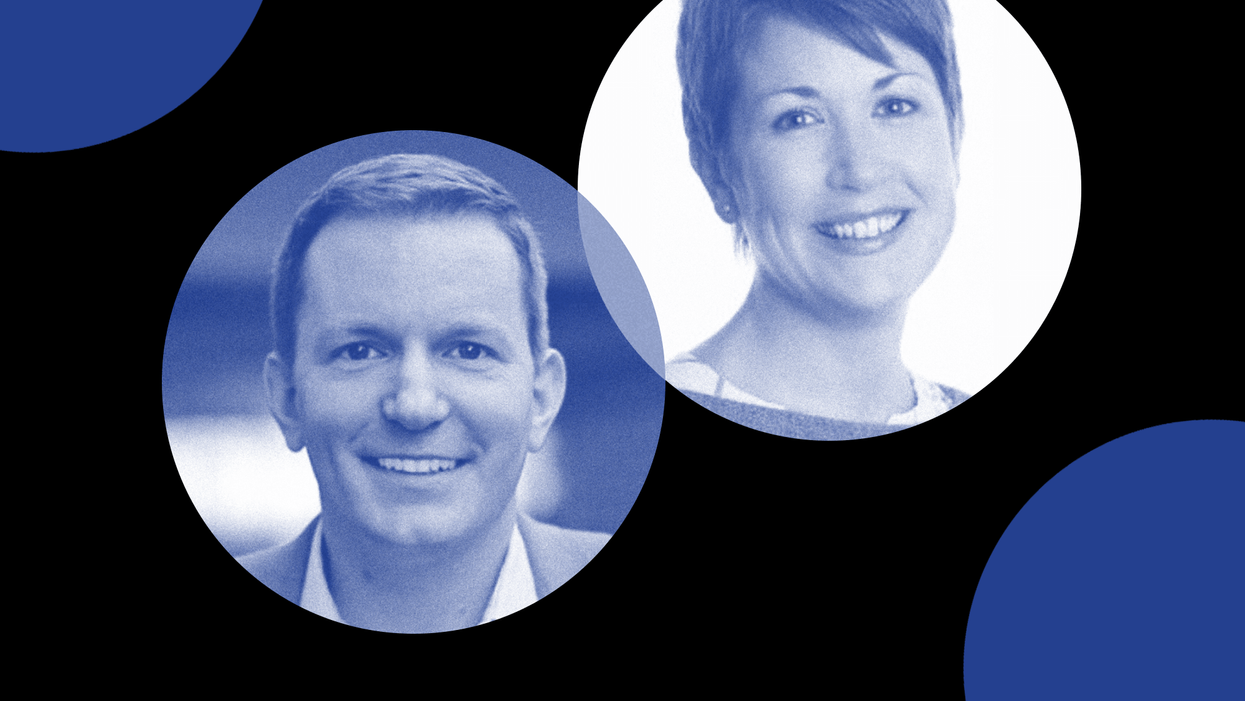
Scientists Jason Schrum and Kerry Benenato solved crucial challenges in mRNA vaccine development.
In early 2020, Moderna Inc. was a barely-known biotechnology company with an unproven approach. It wanted to produce messenger RNA molecules to carry instructions into the body, teaching it to ward off disease. Experts doubted the Boston-based company would meet success.
Today, Moderna is a pharmaceutical power thanks to its success developing an effective Covid-19 vaccine. The company is worth $124 billion, more than giants including GlaxoSmithKline and Sanofi, and evidence has emerged that Moderna's shots are more protective than those produced by Pfizer-BioNTech and other vaccine makers. Pressure is building on the company to deliver more of its doses to people around the world, especially in poorer countries, and Moderna is working on vaccines against other pathogens, including Zika, influenza and cytomegalovirus.
But Moderna encountered such difficulties over the course of its eleven-year history that some executives worried it wouldn't survive. Two unlikely scientists helped save the company. Their breakthroughs paved the way for Moderna's Covid-19 shots but their work has never been publicized nor have their contributions been properly appreciated.
Derrick Rossi, a scientist at MIT, and Noubar Afeyan, a Cambridge-based investor, launched Moderna in September 2010. Their idea was to create mRNA molecules capable of delivering instructions to the body's cells, directing them to make proteins to heal ailments and cure disease. Need a statin, immunosuppressive, or other drug or vaccine? Just use mRNA to send a message to the body's cells to produce it. Rossi and Afeyan were convinced injecting mRNA into the body could turn it into its own laboratory, generating specific medications or vaccines as needed.
At the time, the notion that one might be able to teach the body to make proteins bordered on heresy. Everyone knew mRNA was unstable and set off the body's immune system on its way into cells. But in the late 2000's, two scientists at the University of Pennsylvania, Katalin Karikó and Drew Weissman, had figured out how to modify mRNA's chemical building blocks so the molecule could escape the notice of the immune system and enter the cell. Rossi and Afeyan couldn't convince the University of Pennsylvania to license Karikó and Weissman's patent, however, stymying Moderna's early ambitions. At the same time, the Penn scientists' technique seemed more applicable to an academic lab than a biotech company that needed to produce drugs or shots consistently and in bulk. Rossi and Afeyan's new company needed their own solution to help mRNA evade the body's defenses.
Some of Moderna's founders doubted Schrum could find success and they worried if their venture was doomed from the start.
The Scientist Who Modified mRNA: Jason Schrum
In 2010, Afeyan's firm subleased laboratory space in the basement of another Cambridge biotech company to begin scientific work. Afeyan chose a young scientist on his staff, Jason Schrum, to be Moderna's first employee, charging him with getting mRNA into cells without relying on Karikó and Weissman's solutions.
Schrum seemed well suited for the task. Months earlier, he had received a PhD in biological chemistry at Harvard University, where he had focused on nucleotide chemistry. Schrum even had the look of someone who might do big things. The baby-faced twenty-eight-year-old favored a relaxed, start-up look: khakis, button-downs, and Converse All-Stars.
Schrum felt immediate strain, however. He hadn't told anyone, but he was dealing with intense pain in his hands and joints, a condition that later would be diagnosed as degenerative arthritis. Soon Schrum couldn't bend two fingers on his left hand, making lab work difficult. He joined a drug trial, but the medicine proved useless. Schrum tried corticosteroid injections and anti-inflammatory drugs, but his left hand ached, restricting his experiments.
"It just wasn't useful," Schrum says, referring to his tender hand.*
He persisted, nonetheless. Each day in the fall of 2010, Schrum walked through double air-locked doors into a sterile "clean room" before entering a basement laboratory, in the bowels of an office in Cambridge's Kendall Square neighborhood, where he worked deep into the night. Schrum searched for potential modifications of mRNA nucleosides, hoping they might enable the molecule to produce proteins. Like all such rooms, there were no windows, so Schrum had to check a clock to know if it was day or night. A colleague came to visit once in a while, but most of the time, Schrum was alone.
Some of Moderna's founders doubted Schrum could find success and they worried if their venture was doomed from the start. An established MIT scientist turned down a job with the start-up to join pharmaceutical giant Novartis, dubious of Moderna's approach. Colleagues wondered if mRNA could produce proteins, at least on a consistent basis.
As Schrum began testing the modifications in January 2011, he made an unexpected discovery. Karikó and Weissman saw that by turned one of the building blocks for mRNA, a ribonucleoside called uridine, into a slightly different form called pseudouridine, the cell's immune system ignored the mRNA and the molecule avoided an immune response. After a series of experiments in the basement lab, Schrum discovered that a variant of pseudouridine called N1- methyl-pseudouridine did an even better job reducing the cell's innate immune response. Schrum's nucleoside switch enabled even higher protein production than Karikó and Weissman had generated, and Schrum's mRNAs lasted longer than either unmodified molecules or the modified mRNA the Penn academics had used, startling the young researcher. Working alone in a dreary basement and through intense pain, he had actually improved on the Penn professors' work.
Years later, Karikó and Weissman who would win acclaim. In September 2021, the scientists were awarded the Lasker-DeBakey Clinical Medical Research Award. Some predict they eventually will win a Nobel prize. But it would be Schrum's innovation that would form the backbone of both Moderna and Pfizer-BioNTech's Covid-19 vaccine, not the chemical modifications that Karikó and Weissman developed. For Schrum, necessity had truly been the mother of invention.
The Scientist Who Solved Delivery: Kerry Benenato
For several years, Moderna would make slow progress developing drugs to treat various diseases. Eventually, the company decided that mRNA was likely better suited for vaccines. By 2017, Moderna and the National Institutes of Health were discussing working together to develop mRNA–based vaccines, a partnership that buoyed Moderna's executives. There remained a huge obstacle in Moderna's way, however. It was up to Kerry Benenato to find a solution.
Benenato received an early hint of the hurdle in front of her three years earlier, when the organic chemist was first hired. When a colleague gave her a company tour, she was introduced to Moderna's chief scientific officer, Joseph Bolen, who seemed unusually excited to meet her.
"Oh, great!" Bolen said with a smile. "She's the one who's gonna solve delivery."
Bolen gave a hearty laugh and walked away, but Benenato detected seriousness in his quip.
Solve delivery?
It was a lot to expect from a 37-year-old scientist already dealing with insecurities and self-doubt. Benenato was an accomplished researcher who most recently had worked at AstraZeneca after completing post-doctoral studies at Harvard University. Despite her impressive credentials, Benenato battled a lack of confidence that sometimes got in her way. Performance reviews from past employers had been positive, but they usually produced similar critiques: Be more vocal. Do a better job advocating for your ideas. Give us more, Kerry.
Benenato was petite and soft-spoken. She sometimes stuttered or relied on "ums" and "ahs" when she became nervous, especially in front of groups, part of why she sometimes didn't feel comfortable speaking up.
"I'm an introvert," she says. "Self-confidence is something that's always been an issue."
To Benenato, Moderna's vaccine approach seemed promising—the team was packaging mRNAs in microscopic fatty-acid compounds called lipid nanoparticles, or LNPs, that protected the molecules on their way into cells. Moderna's shots should have been producing ample and long-lasting proteins. But the company's scientists were alarmed—they were injecting shots deep into the muscle of mice, but their immune systems were mounting spirited responses to the foreign components of the LNPs, which had been developed by a Canadian company.
This toxicity was a huge issue: A vaccine or drug that caused sharp pain and awful fevers wasn't going to prove very popular. The Moderna team was in a bind: Its mRNA had to be wrapped in the fatty nanoparticles to have a chance at producing plentiful proteins, but the body wasn't tolerating the microscopic encasements, especially upon repeated dosing.
The company's scientists had done everything they could to try to make the molecule's swathing material disappear soon after entering the cells, in order to avoid the unfortunate side effects, such as chills and headaches, but they weren't making headway. Frustration mounted. Somehow, the researchers had to find a way to get the encasements—made of little balls of fat, cholesterol, and other substances—to deliver their payload mRNA and then quickly vanish, like a parent dropping a teenager off at a party, to avoid setting off the immune system in unpleasant ways, even as the RNA and the proteins the molecule created stuck around.
Benenato wasn't entirely shocked by the challenges Moderna was facing. One of the reasons she had joined the upstart company was to help develop its delivery technology. She just didn't realize how pressing the issue was, or how stymied the researchers had become. Benenato also didn't know that Moderna board members were among those most discouraged by the delivery issue. In meetings, some of them pointed out that pharmaceutical giants like Roche Holding and Novartis had worked on similar issues and hadn't managed to develop lipid nanoparticles that were both effective and well tolerated by the body. Why would Moderna have any more luck?
Stephen Hoge insisted the company could yet find a solution.
"There's no way the only innovations in LNP are going to come from some academics and a small Canadian company," insisted Hoge, who had convinced the executives that hiring Benenato might help deliver an answer.
Benenato realized that while Moderna might have been a hot Boston-area start- up, it wasn't set up to do the chemistry necessary to solve their LNP problem. Much of its equipment was old or secondhand, and it was the kind used to tinker with mRNAs, not lipids.
"It was scary," she says.
When Benenato saw the company had a nuclear magnetic resonance spectrometer, which allows chemists to see the molecular structure of material, she let out a sigh of relief. Then Benenato inspected the machine and realized it was a jalopy. The hulking, aging instrument had been decommissioned and left behind by a previous tenant, too old and banged up to bring with them.
Benenato began experimenting with different chemical changes for Moderna's LNPs, but without a working spectrometer she and her colleagues had to have samples ready by noon each day, so they could be picked up by an outside company that would perform the necessary analysis. After a few weeks, her superiors received an enormous bill for the outsourced work and decided to pay to get the old spectrometer running again.
After months of futility, Benenato became impatient. An overachiever who could be hard on herself, she was eager to impress her new bosses. Benenato felt pressure outside the office, as well. She was married with a preschool-age daughter and an eighteen-month-old son. In her last job, Benenato's commute had been a twenty-minute trip to Astra-Zeneca's office in Waltham, outside Boston; now she was traveling an hour to Moderna's Cambridge offices. She became anxious—how was she going to devote the long hours she realized were necessary to solve their LNP quandary while providing her children proper care? Joining Moderna was beginning to feel like a possible mistake.
She turned to her husband and father for help. They reminded her of the hard work she had devoted to establishing her career and said it would be a shame if she couldn't take on the new challenge. Benenato's husband said he was happy to stay home with the kids, alleviating some of her concerns.
Back in the office, she got to work. She wanted to make lipids that were easier for the body to chop into smaller pieces, so they could be eliminated by the body's enzymes. Until then, Moderna, like most others, relied on all kinds of complicated chemicals to hold its LNP packaging together. They weren't natural, though, so the body was having a hard time breaking them down, causing the toxicity.
Benenato began experimenting with simpler chemicals. She inserted "ester bonds"—compounds referred to in chemical circles as "handles" because the body easily grabs them and breaks them apart. Ester bonds had two things going for them: They were strong enough to help ensure the LNP remained stable, acting much like a drop of oil in water, but they also gave the body's enzymes something to target and break down as soon as the LNP entered the cell, a way to quickly rid the body of the potentially toxic LNP components. Benenato thought the inclusion of these chemicals might speed the elimination of the LNP delivery material.
This idea, Benenato realized, was nothing more than traditional, medicinal chemistry. Most people didn't use ester bonds because they were pretty unsophisticated. But, hey, the tricky stuff wasn't working, so Benenato thought she'd see if the simple stuff worked.
Benenato also wanted to try to replace a group of unnatural chemicals in the LNP that was contributing to the spirited and unwelcome response from the immune system. Benenato set out to build a new and improved chemical combination. She began with ethanolamine, a colorless, natural chemical, an obvious start for any chemist hoping to build a more complex chemical combination. No one relied on ethanolamine on its own.
Benenato was curious, though. What would happen if she used just these two simple modifications to the LNP: ethanolamine with the ester bonds? Right away, Benenato noticed her new, super-simple compound helped mRNA create some protein in animals. It wasn't much, but it was a surprising and positive sign. Benenato spent over a year refining her solution, testing more than one hundred variations, all using ethanolamine and ester bonds, showing improvements with each new version of LNP. After finishing her 102nd version of the lipid molecule, which she named SM102, Benenato was confident enough in her work to show it to Hoge and others.
They immediately got excited. The team kept tweaking the composition of the lipid encasement. In 2017, they wrapped it around mRNA molecules and injected the new combination in mice and then monkeys. They saw plentiful, potent proteins were being produced and the lipids were quickly being eliminated, just as Benenato and her colleagues had hoped. Moderna had its special sauce.
That year, Benenato was asked to deliver a presentation to Stephane Bancel, Moderna's chief executive, Afeyan, and Moderna's executive committee to explain why it made sense to use the new, simpler LNP formulation for all its mRNA vaccines. She still needed approval from the executives to make the change. Ahead of the meeting, she was apprehensive, as some of her earlier anxieties returned. But an unusual calm came over her as she began speaking to the group. Benenato explained how experimenting with basic, overlooked chemicals had led to her discovery.
She said she had merely stumbled onto the company's solution, though her bosses understood the efforts that had been necessary for the breakthrough. The board complimented her work and agreed with the idea of switching to the new LNP. Benenato beamed with pride.
"As a scientist, serendipity has been my best friend," she told the executives.
Over the next few years, Benenato and her colleagues would improve on their methods and develop even more tolerable and potent LNP encasement for mRNA molecules. Their work enabled Moderna to include higher doses of vaccine in its shots. In early 2020, Moderna developed Covid-19 shots that included 100 micrograms of vaccine, compared with 30 micrograms in the Pfizer-BioNTech vaccine. That difference appears to help the Moderna vaccine generate higher titers and provide more protection.
"You set out in a career in drug discovery to want to make a difference," Benenato says. "Seeing it come to reality has been surreal and emotional."
Editor's Note: This essay is excerpted from A SHOT TO SAVE THE WORLD: The Inside Story of the Life-or-Death Race for a COVID-19 Vaccine by Gregory Zuckerman, now on sale from Portfolio/Penguin.
*Jason Schrum's arthritis is now in complete remission, thanks to Humira (adalimumab), a TNF-alpha blocker.
Health breakthroughs of 2022 that should have made bigger news
Nine experts break down the biggest biotech and health breakthroughs that didn't get the attention they deserved in 2022.
As the world has attempted to move on from COVID-19 in 2022, attention has returned to other areas of health and biotech with major regulatory approvals such as the Alzheimer's drug lecanemab – which can slow the destruction of brain cells in the early stages of the disease – being hailed by some as momentous breakthroughs.
This has been a year where psychedelic medicines have gained the attention of mainstream researchers with a groundbreaking clinical trial showing that psilocybin treatment can help relieve some of the symptoms of major depressive disorder. And with messenger RNA (mRNA) technology still very much capturing the imagination, the readouts of cancer vaccine trials have made headlines around the world.
But at the same time there have been vital advances which will likely go on to change medicine, and yet have slipped beneath the radar. I asked nine forward-thinking experts on health and biotech about the most important, but underappreciated, breakthrough of 2022.
Their descriptions, below, were lightly edited by Leaps.org for style and format.
New drug targets for Alzheimer’s disease
Professor Julie Williams, Director, Dementia Research Institute, Cardiff University
Genetics has changed our view of Alzheimer’s disease in the last five to six years. The beta amyloid hypothesis has dominated Alzheimer’s research for a long time, but there are multiple components to this complex disease, of which getting rid of amyloid plaques is one, but it is not the whole story. In April 2022, Nature published a paper which is the culmination of a decade’s worth of work - groups all over the world working together to identify 75 genes associated with risk of developing Alzheimer’s. This provides us with a roadmap for understanding the disease mechanisms.
For example, it is showing that there is something different about the immune systems of people who develop Alzheimer’s disease. There is something different about the way they process lipids in the brain, and very specific processes of how things travel through cells called endocytosis. When it comes to immunity, it indicates that the complement system is affecting whether synapses, which are the connections between neurons, get eliminated or not. In Alzheimer’s this process is more severe, so patients are losing more synapses, and this is correlated with cognition.
The genetics also implicates very specific tissues like microglia, which are the housekeepers in the brain. One of their functions is to clear away beta amyloid, but they also prune and nibble away at parts of the brain that are indicated to be diseased. If you have these risk genes, it seems that you are likely to prune more tissue, which may be part of the cell death and neurodegeneration that we observe in Alzheimer’s patients.
Genetics is telling us that we need to be looking at multiple causes of this complex disease, and we are doing that now. It is showing us that there are a number of different processes which combine to push patients into a disease state which results in the death of connections between nerve cells. These findings around the complement system and other immune-related mechanisms are very interesting as there are already drugs which are available for other diseases which could be repurposed in clinical trials. So it is really a turning point for us in the Alzheimer’s disease field.
Preventing Pandemics with Organ-Tissue Equivalents
Anthony Atala, Director of the Wake Forest Institute for Regenerative Medicine
COVID-19 has shown us that we need to be better prepared ahead of future pandemics and have systems in place where we can quickly catalogue a new virus and have an idea of which treatment agents would work best against it.
At Wake Forest Institute, our scientists have developed what we call organ-tissue equivalents. These are miniature tissues and organs, created using the same regenerative medicine technologies which we have been using to create tissues for patients. For example, if we are making a miniature liver, we will recreate this structure using the six different cell types you find in the liver, in the right proportions, and then the right extracellular matrix which holds the structure together. You're trying to replicate all the characteristics of the liver, but just in a miniature format.
We can now put these organ-tissue equivalents in a chip-like device, where we can expose them to different types of viral infections, and start to get a realistic idea of how the human body reacts to these viruses. We can use artificial intelligence and machine learning to map the pathways of the body’s response. This will allow us to catalogue known viruses far more effectively, and begin storing information on them.
Powering Deep Brain Stimulators with Breath
Islam Mosa, Co-Founder and CTO of VoltXon
Deep brain stimulation (DBS) devices are becoming increasingly common with 150,000 new devices being implanted every year for people with Parkinson’s disease, but also psychiatric conditions such as treatment-resistant depression and obsessive-compulsive disorders. But one of the biggest limitations is the power source – I call DBS devices energy monsters. While cardiac pacemakers use similar technology, their batteries last seven to ten years, but DBS batteries need changing every two to three years. This is because they are generating between 60-180 pulses per second.
Replacing the batteries requires surgery which costs a lot of money, and with every repeat operation comes a risk of infection, plus there is a lot of anxiety on behalf of the patient that the battery is running out.
My colleagues at the University of Connecticut and I, have developed a new way of charging these devices using the person’s own breathing movements, which would mean that the batteries never need to be changed. As the patient breathes in and out, their chest wall presses on a thin electric generator, which converts that movement into static electricity, charging a supercapacitor. This discharges the electricity required to power the DBS device and send the necessary pulses to the brain.
So far it has only been tested in a simulated pig, using a pig lung connected to a pump, but there are plans now to test it in a real animal, and then progress to clinical trials.
Smartwatches for Disease Detection
Jessilyn Dunn, Assistant Professor in Duke Biomedical Engineering
A group of researchers recently showed that digital biomarkers of infection can reveal when someone is sick, often before they feel sick. The team, which included Duke biomedical engineers, used information from smartwatches to detect Covid-19 cases five to 10 days earlier than diagnostic tests. Smartwatch data included aspects of heart rate, sleep quality and physical activity. Based on this data, we developed an algorithm to decide which people have the most need to take the diagnostic tests. With this approach, the percent of tests that come back positive are about four- to six-times higher, depending on which factors we monitor through the watches.
Our study was one of several showing the value of digital biomarkers, rather than a single blockbuster paper. With so many new ideas and technologies coming out around Covid, it’s hard to be that signal through the noise. More studies are needed, but this line of research is important because, rather than treat everyone as equally likely to have an infectious disease, we can use prior knowledge from smartwatches. With monkeypox, for example, you've got many more people who need to be tested than you have tests available. Information from the smartwatches enables you to improve how you allocate those tests.
Smartwatch data could also be applied to chronic diseases. For viruses, we’re looking for information about anomalies – a big change point in people’s health. For chronic diseases, it’s more like a slow, steady change. Our research lays the groundwork for the signals coming from smartwatches to be useful in a health setting, and now it’s up to us to detect more of these chronic cases. We want to go from the idea that we have this single change point, like a heart attack or stroke, and focus on the part before that, to see if we can detect it.
A Vaccine For RSV
Norbert Pardi, Vaccines Group Lead, Penn Institute for RNA Innovation, University of Pennsylvania
Scientists have long been trying to develop a vaccine for respiratory syncytial virus (RSV), and it looks like Pfizer are closing in on this goal, based on the latest clinical trial data in newborns which they released in November. Pfizer have developed a protein-based vaccine against the F protein of RSV, which they are giving to pregnant women. It turns out that it induces a robust immune response after the administration of a single shot and it seems to be highly protective in newborns. The efficacy was over 80% after 90 days, so it protected very well against severe disease, and even though this dropped a little after six month, it was still pretty high.
I think this has been a very important breakthrough, and very timely at the moment with both COVID-19, influenza and RSV circulating, which just shows the importance of having a vaccine which works well in both the very young and the very old.
The road to an RSV vaccine has also illustrated the importance of teamwork in 21st century vaccine development. You need people with different backgrounds to solve these challenges – microbiologists, immunologists and structural biologists working together to understand how viruses work, and how our immune system induces protective responses against certain viruses. It has been this kind of teamwork which has yielded the findings that targeting the prefusion stabilized form of the F protein in RSV induces much stronger and highly protective immune responses.
Gene therapy shows its potential
Nicole Paulk, Assistant Professor of Gene Therapy at the University of California, San Francisco
The recent US Food and Drug Administration (FDA) approval of Hemgenix, a gene therapy for hemophilia B, is big for a lot of reasons. While hemophilia is absolutely a rare disease, it is astronomically more common than the first two approvals – Luxturna for RPE65-meidated inherited retinal dystrophy and Zolgensma for spinal muscular atrophy - so many more patients will be treated with this. In terms of numbers of patients, we are now starting to creep up into things that are much more common, which is a huge step in terms of our ability to scale the production of an adeno-associated virus (AAV) vector for gene therapy.
Hemophilia is also a really special patient population because this has been the darling indication for AAV gene therapy for the last 20 to 30 years. AAV trafficks to the liver so well, it’s really easy for us to target the tissues that we want. If you look at the numbers, there have been more gene therapy scientists working on hemophilia than any other condition. There have just been thousands and thousands of us working on gene therapy indications for the last 20 or 30 years, so to see the first of these approvals make it, feels really special.
I am sure it is even more special for the patients because now they have a choice – do I want to stay on my recombinant factor drug that I need to take every day for the rest of my life, or right now I could get a one-time infusion of this virus and possibly experience curative levels of expression for the rest of my life. And this is just the first one for hemophilia, there’s going to end up being a dozen gene therapies within the next five years, targeted towards different hemophilias.
Every single approval is momentous for the entire field because it gets investors excited, it gets companies and physicians excited, and that helps speed things up. Right now, it's still a challenge to produce enough for double digit patients. But with more interest comes the experiments and trials that allow us to pick up the knowledge to scale things up, so that we can go after bigger diseases like diabetes, congestive heart failure, cancer, all of these much bigger afflictions.
Treating Thickened Hearts
John Spertus, Professor in Metabolic and Vascular Disease Research, UMKC School of Medicine
Hypertrophic cardiomyopathy (HCM) is a disease that causes your heart muscle to enlarge, and the walls of your heart chambers thicken and reduce in size. Because of this, they cannot hold as much blood and may stiffen, causing some sufferers to experience progressive shortness of breath, fatigue and ultimately heart failure.
So far we have only had very crude ways of treating it, using beta blockers, calcium channel blockers or other medications which cause the heart to beat less strongly. This works for some patients but a lot of time it does not, which means you have to consider removing part of the wall of the heart with surgery.
Earlier this year, a trial of a drug called mavacamten, became the first study to show positive results in treating HCM. What is remarkable about mavacamten is that it is directed at trying to block the overly vigorous contractile proteins in the heart, so it is a highly targeted, focused way of addressing the key problem in these patients. The study demonstrated a really large improvement in patient quality of life where they were on the drug, and when they went off the drug, the quality of life went away.
Some specialists are now hypothesizing that it may work for other cardiovascular diseases where the heart either beats too strongly or it does not relax well enough, but just having a treatment for HCM is a really big deal. For years we have not been very aggressive in identifying and treating these patients because there have not been great treatments available, so this could lead to a new era.
Regenerating Organs
David Andrijevic, Associate Research Scientist in neuroscience at Yale School of Medicine
As soon as the heartbeat stops, a whole chain of biochemical processes resulting from ischemia – the lack of blood flow, oxygen and nutrients – begins to destroy the body’s cells and organs. My colleagues and I at Yale School of Medicine have been investigating whether we can recover organs after prolonged ischemia, with the main goal of expanding the organ donor pool.
Earlier this year we published a paper in which we showed that we could use technology to restore blood circulation, other cellular functions and even heart activity in pigs, one hour after their deaths. This was done using a perfusion technology to substitute heart, lung and kidney function, and deliver an experimental cell protective fluid to these organs which aimed to stop cell death and aid in the recovery.
One of the aims of this technology is that it can be used in future to lengthen the time window for recovering organs for donation after a person has been declared dead, a logistical hurdle which would allow us to substantially increase the donor pool. We might also be able to use this cell protective fluid in studies to see if it can help people who have suffered from strokes and myocardial infarction. In future, if we managed to achieve an adequate brain recovery – and the brain, out of all the organs, is the most susceptible to ischemia – this might also change some paradigms in resuscitation medicine.
Antibody-Drug Conjugates for Cancer
Yosi Shamay, Cancer Nanomedicine and Nanoinformatics researcher at the Technion Israel Institute of Technology
For the past four or five years, antibody-drug conjugates (ADCs) - a cancer drug where you have an antibody conjugated to a toxin - have been used only in patients with specific cancers that display high expression of a target protein, for example HER2-positive breast cancer. But in 2022, there have been clinical trials where ADCs have shown remarkable results in patients with low expression of HER2, which is something we never expected to see.
In July 2022, AstraZeneca published the results of a clinical trial, which showed that an ADC called trastuzumab deruxtecan can offer a very big survival benefit to breast cancer patients with very little expression of HER2, levels so low that they would be borderline undetectable for a pathologist. They got a strong survival signal for patients with very aggressive, metastatic disease.
I think this is very interesting and important because it means that it might pave the way to include more patients in clinical trials looking at ADCs for other cancers, for example lymphoma, colon cancer, lung cancers, even if they have low expression of the protein target. It also holds implications for CAR-T cells - where you genetically engineer a T cell to attack the cancer - because the concept is very similar. If we now know that an ADC can have a survival benefit, even in patients with very low target expression, the same might be true for T cells.
Look back further: Breakthroughs of 2021
https://leaps.org/6-biotech-breakthroughs-of-2021-that-missed-the-attention-they-deserved/
Repairing Cells and Longevity Myths with Dr. Charles Brenner
Charles Brenner, a leading biochemist at City of Hope National Medical Center in L.A., discovered a vitamin precursor called NR that seems to enable repair of cellular damage that happens as we get older.
Meet Charles Brenner, the Longevity Skeptic. Brenner, a leading biochemist at City of Hope National Medical Center in L.A., has been attending the largest longevity conferences with one main purpose: to point out that some of the other speakers are full of it.
Brenner is "throwing cold water" on several scientists in the field of aging, accusing them of hyping various fountains of youth, despite limited evidence for these therapies.
In this podcast episode, Brenner sat down with Leaps.org to discuss his groundbreaking work on metabolism and his efforts to counter what he considers to be bad science.
Listen on Apple | Listen on Spotify | Listen on Stitcher | Listen on Amazon | Listen on Google
In addition to bringing his candor to conferences, Brenner is applying it in academic journals, publishing a paper in September, "A Science-Based Review of the World's Best-Selling Book on Aging," in which he pans the author of this bestseller, David Sinclair, a Harvard biologist, for talking up the potential for humans to live far past 100. These aspirations may sound nice, but they're not backed by science, Brenner says. He's had high-profile debates online with Sinclair and Aubrey de Grey, a prominent biomedical gerontologist.
Meanwhile, in his own lab work, Brenner is credited with identifying a vitamin precursor called NR that seems to enable repair of cellular damage that happens as we get older - a major discovery that he's helped turn into a supplement, commercialized with a company called ChromaDex.
Whether it's possible to extend human lifespan is a pressing question as investments in longevity startups are projected to increase from $40 billion to $600 billion over the next three years. The field of biological aging seems split on the question of whether "anti-aging" therapies can significantly lengthen our natural lifespans, as Sinclair believes. Brenner, Morgan Levine of Altos Labs and Matt Kaeberlein of the University of Washington have argued that the only realistic goal is to extend one's window of healthy years, or healthspan, rather than trying to break the biological ceiling of our species.
Brenner is an intriguing figure in these debates. Although he’s been introduced in public appearances as a longevity skeptic, he calls himself an optimist.
Links:
Charles Brenner, City of Hope
Charles Brenner on Twitter
Charles Brenner's debate with Aubrey de Grey
Brenner's paper, "A Science-Based Review of the World's Best-Selling Book on Aging"
Peter Attia's recent blog on NR supplements
Brad Stanfield's recent reaction to "David Sinclair vs Charles Brenner"
ChromaDex NR supplement