Indigenous wisdom plus honeypot ants could provide new antibiotics
Lina Zeldovich has written about science, medicine and technology for Popular Science, Smithsonian, National Geographic, Scientific American, Reader’s Digest, the New York Times and other major national and international publications. A Columbia J-School alumna, she has won several awards for her stories, including the ASJA Crisis Coverage Award for Covid reporting, and has been a contributing editor at Nautilus Magazine. In 2021, Zeldovich released her first book, The Other Dark Matter, published by the University of Chicago Press, about the science and business of turning waste into wealth and health. You can find her on http://linazeldovich.com/ and @linazeldovich.
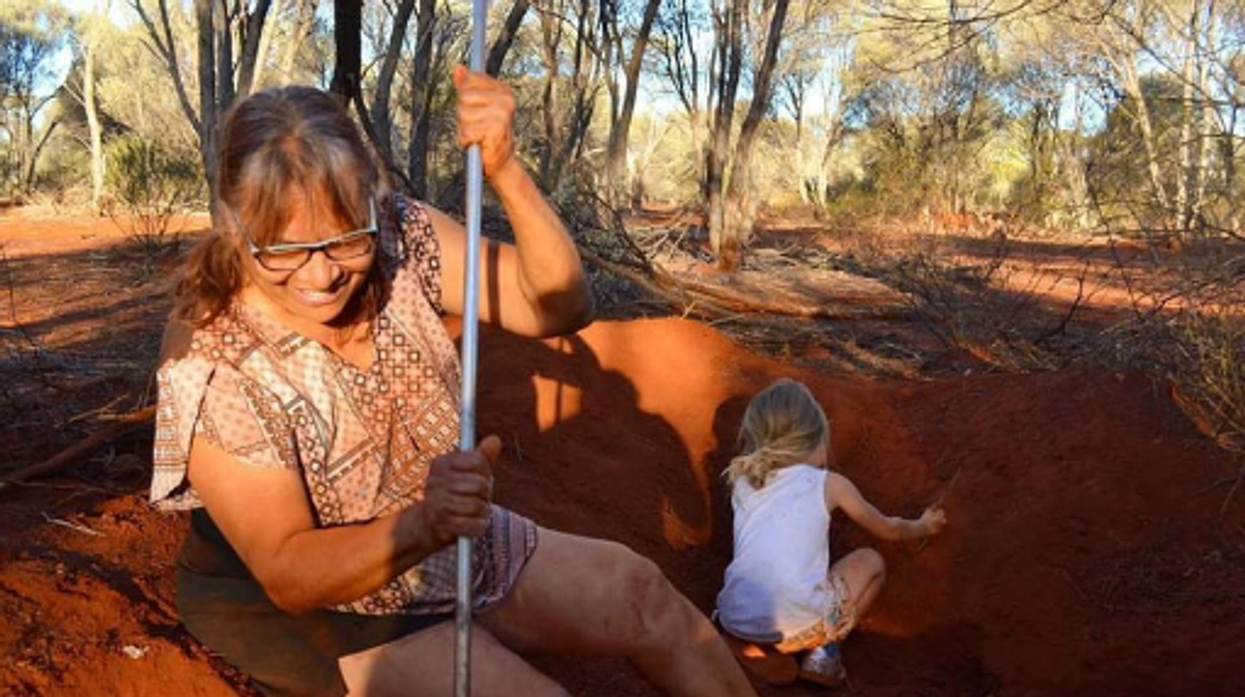
Indigenous people in Australia dig pits next to a honeypot colony. Scientists think the honey can be used to make new antimicrobial drugs.
For generations, the Indigenous Tjupan people of Australia enjoyed the sweet treat of honey made by honeypot ants. As a favorite pastime, entire families would go searching for the underground colonies, first spotting a worker ant and then tracing it to its home. The ants, which belong to the species called Camponotus inflatus, usually build their subterranean homes near the mulga trees, Acacia aneura. Having traced an ant to its tree, it would be the women who carefully dug a pit next to a colony, cautious not to destroy the entire structure. Once the ant chambers were exposed, the women would harvest a small amount to avoid devastating the colony’s stocks—and the family would share the treat.
The Tjupan people also knew that the honey had antimicrobial properties. “You could use it for a sore throat,” says Danny Ulrich, a member of the Tjupan nation. “You could also use it topically, on cuts and things like that.”
These hunts have become rarer, as many of the Tjupan people have moved away and, up until now, the exact antimicrobial properties of the ant honey remained unknown. But recently, scientists Andrew Dong and Kenya Fernandes from the University of Sydney, joined Ulrich, who runs the Honeypot Ants tours in Kalgoorlie, a city in Western Australia, on a honey-gathering expedition. Afterwards, they ran a series of experiments analyzing the honey’s antimicrobial activity—and confirmed that the Indigenous wisdom was true. The honey was effective against Staphylococcus aureus, a common pathogen responsible for sore throats, skin infections like boils and sores, and also sepsis, which can result in death. Moreover, the honey also worked against two species of fungi, Cryptococcus and Aspergillus, which can be pathogenic to humans, especially those with suppressed immune systems.
In the era of growing antibiotic resistance and the rising threat of pathogenic fungi, these findings may help scientists identify and make new antimicrobial compounds. “Natural products have been honed over thousands and millions of years by nature and evolution,” says Fernandes. “And some of them have complex and intricate properties that make them really important as potential new antibiotics. “
In an era of growing resistance to antibiotics and new threats of fungi infections, the latest findings about honeypot ants are helping scientists identify new antimicrobial drugs.
Danny Ulrich
Bee honey is also known for its antimicrobial properties, but bees produce it very differently than the ants. Bees collect nectar from flowers, which they regurgitate at the hive and pack into the hexagonal honeycombs they build for storage. As they do so, they also add into the mix an enzyme called glucose oxidase produced by their glands. The enzyme converts atmospheric oxygen into hydrogen peroxide, a reactive molecule that destroys bacteria and acts as a natural preservative. After the bees pack the honey into the honeycombs, they fan it with their wings to evaporate the water. Once a honeycomb is full, the bees put a beeswax cover on it, where it stays well-preserved thanks to the enzymatic action, until the bees need it.
Less is known about the chemistry of ants’ honey-making. Similarly to bees, they collect nectar. They also collect the sweet sap of the mulga tree. Additionally, they also “milk” the aphids—small sap-sucking insects that live on the tree. When ants tickle the aphids with their antennae, the latter release a sweet substance, which the former also transfer to their colonies. That’s where the honey management difference becomes really pronounced. The ants don’t build any kind of structures to store their honey. Instead, they store it in themselves.
The workers feed their harvest to their fellow ants called repletes, stuffing them up to the point that their swollen bellies outgrow the ants themselves, looking like amber-colored honeypots—hence the name. Because of their size, repletes don’t move, but hang down from the chamber’s ceiling, acting as living feedstocks. When food becomes scarce, they regurgitate their reserves to their colony’s brethren. It’s not clear whether the repletes die afterwards or can be restuffed again. “That's a good question,” Dong says. “After they've been stretched, they can't really return to exactly the same shape.”
These replete ants are the “treat” the Tjupan women dug for. Once they saw the round-belly ants inside the chambers, they would reach in carefully and get a few scoops of them. “You see a lot of honeypot ants just hanging on the roof of the little openings,” says Ulrich’s mother, Edie Ulrich. The women would share the ants with family members who would eat them one by one. “They're very delicate,” shares Edie Ulrich—you have to take them out carefully, so they don’t accidentally pop and become a wasted resource. “Because you’d lose all this precious honey.”
Dong stumbled upon the honeypot ants phenomenon because he was interested in Indigenous foods and went on Ulrich’s tour. He quickly became fascinated with the insects and their role in the Indigenous culture. “The honeypot ants are culturally revered by the Indigenous people,” he says. Eventually he decided to test out the honey’s medicinal qualities.
The researchers were surprised to see that even the smallest, eight percent concentration of honey was able to arrest the growth of S. aureus.
To do this, the two scientists first diluted the ant honey with water. “We used something called doubling dilutions, which means that we made 32 percent dilutions, and then we halve that to 16 percent and then we half that to eight percent,” explains Fernandes. The goal was to obtain as much results as possible with the meager honey they had. “We had very, very little of the honeypot ant honey so we wanted to maximize the spectrum of results we can get without wasting too much of the sample.”
After that, the researchers grew different microbes inside a nutrient rich broth. They added the broth to the different honey dilutions and incubated the mixes for a day or two at the temperature favorable to the germs’ growth. If the resulting solution turned turbid, it was a sign that the bugs proliferated. If it stayed clear, it meant that the honey destroyed them. The researchers were surprised to see that even the smallest, eight percent concentration of honey was able to arrest the growth of S. aureus. “It was really quite amazing,” Fernandes says. “Eight milliliters of honey in 92 milliliters of water is a really tiny amount of honey compared to the amount of water.”
Similar to bee honey, the ants’ honey exhibited some peroxide antimicrobial activity, researchers found, but given how little peroxide was in the solution, they think the honey also kills germs by a different mechanism. “When we measured, we found that [the solution] did have some hydrogen peroxide, but it didn't have as much of it as we would expect based on how active it was,” Fernandes says. “Whether this hydrogen peroxide also comes from glucose oxidase or whether it's produced by another source, we don't really know,” she adds. The research team does have some hypotheses about the identity of this other germ-killing agent. “We think it is most likely some kind of antimicrobial peptide that is actually coming from the ant itself.”
The honey also has a very strong activity against the two types of fungi, Cryptococcus and Aspergillus. Both fungi are associated with trees and decaying leaves, as well as in the soils where ants live, so the insects likely have evolved some natural defense compounds, which end up inside the honey.
It wouldn’t be the first time when modern medicines take their origin from the natural world or from the indigenous people’s knowledge. The bark of the cinchona tree native to South America contains quinine, a substance that treats malaria. The Indigenous people of the Andes used the bark to quell fever and chills for generations, and when Europeans began to fall ill with malaria in the Amazon rainforest, they learned to use that medicine from the Andean people.
The wonder drug aspirin similarly takes its origin from a bark of a tree—in this case a willow.
Even some anticancer compounds originated from nature. A chemotherapy drug called Paclitaxel, was originally extracted from the Pacific yew trees, Taxus brevifolia. The samples of the Pacific yew bark were first collected in 1962 by researchers from the United States Department of Agriculture who were looking for natural compounds that might have anti-tumor activity. In December 1992, the FDA approved Paclitaxel (brand name Taxol) for the treatment of ovarian cancer and two years later for breast cancer.
In the era when the world is struggling to find new medicines fast enough to subvert a fungal or bacterial pandemic, these discoveries can pave the way to new therapeutics. “I think it's really important to listen to indigenous cultures and to take their knowledge because they have been using these sources for a really, really long time,” Fernandes says. Now we know it works, so science can elucidate the molecular mechanisms behind it, she adds. “And maybe it can even provide a lead for us to develop some kind of new treatments in the future.”
Lina Zeldovich has written about science, medicine and technology for Popular Science, Smithsonian, National Geographic, Scientific American, Reader’s Digest, the New York Times and other major national and international publications. A Columbia J-School alumna, she has won several awards for her stories, including the ASJA Crisis Coverage Award for Covid reporting, and has been a contributing editor at Nautilus Magazine. In 2021, Zeldovich released her first book, The Other Dark Matter, published by the University of Chicago Press, about the science and business of turning waste into wealth and health. You can find her on http://linazeldovich.com/ and @linazeldovich.
Last month, a paper published in Cell by Harvard biologist David Sinclair explored root cause of aging, as well as examining whether this process can be controlled. We talked with Dr. Sinclair about this new research.
What causes aging? In a paper published last month, Dr. David Sinclair, Professor in the Department of Genetics at Harvard Medical School, reports that he and his co-authors have found the answer. Harnessing this knowledge, Dr. Sinclair was able to reverse this process, making mice younger, according to the study published in the journal Cell.
I talked with Dr. Sinclair about his new study for the latest episode of Making Sense of Science. Turning back the clock on mouse age through what’s called epigenetic reprogramming – and understanding why animals get older in the first place – are key steps toward finding therapies for healthier aging in humans. We also talked about questions that have been raised about the research.
Show links:
Dr. Sinclair's paper, published last month in Cell.
Recent pre-print paper - not yet peer reviewed - showing that mice treated with Yamanaka factors lived longer than the control group.
Dr. Sinclair's podcast.
Previous research on aging and DNA mutations.
Dr. Sinclair's book, Lifespan.
Harvard Medical School
Breakthrough therapies are breaking patients' banks. Key changes could improve access, experts say.
Single-treatment therapies are revolutionizing medicine. But insurers and patients wonder whether they can afford treatment and, if they can, whether the high costs are worthwhile.
CSL Behring’s new gene therapy for hemophilia, Hemgenix, costs $3.5 million for one treatment, but helps the body create substances that allow blood to clot. It appears to be a cure, eliminating the need for other treatments for many years at least.
Likewise, Novartis’s Kymriah mobilizes the body’s immune system to fight B-cell lymphoma, but at a cost $475,000. For patients who respond, it seems to offer years of life without the cancer progressing.
These single-treatment therapies are at the forefront of a new, bold era of medicine. Unfortunately, they also come with new, bold prices that leave insurers and patients wondering whether they can afford treatment and, if they can, whether the high costs are worthwhile.
“Most pharmaceutical leaders are there to improve and save people’s lives,” says Jeremy Levin, chairman and CEO of Ovid Therapeutics, and immediate past chairman of the Biotechnology Innovation Organization. If the therapeutics they develop are too expensive for payers to authorize, patients aren’t helped.
“The right to receive care and the right of pharmaceuticals developers to profit should never be at odds,” Levin stresses. And yet, sometimes they are.
Leigh Turner, executive director of the bioethics program, University of California, Irvine, notes this same tension between drug developers that are “seeking to maximize profits by charging as much as the market will bear for cell and gene therapy products and other medical interventions, and payers trying to control costs while also attempting to provide access to medical products with promising safety and efficacy profiles.”
Why Payers Balk
Health insurers can become skittish around extremely high prices, yet these therapies often accompany significant overall savings. For perspective, the estimated annual treatment cost for hemophilia exceeds $300,000. With Hemgenix, payers would break even after about 12 years.
But, in 12 years, will the patient still have that insurer? Therein lies the rub. U.S. payers, are used to a “pay-as-you-go” model, in which the lifetime costs of therapies typically are shared by multiple payers over many years, as patients change jobs. Single treatment therapeutics eliminate that cost-sharing ability.
"As long as formularies are based on profits to middlemen…Americans’ healthcare costs will continue to skyrocket,” says Patricia Goldsmith, the CEO of CancerCare.
“There is a phenomenally complex, bureaucratic reimbursement system that has grown, layer upon layer, during several decades,” Levin says. As medicine has innovated, payment systems haven’t kept up.
Therefore, biopharma companies begin working with insurance companies and their pharmacy benefit managers (PBMs), which act on an insurer’s behalf to decide which drugs to cover and by how much, early in the drug approval process. Their goal is to make sophisticated new drugs available while still earning a return on their investment.
New Payment Models
Pay-for-performance is one increasingly popular strategy, Turner says. “These models typically link payments to evidence generation and clinically significant outcomes.”
A biotech company called bluebird bio, for example, offers value-based pricing for Zynteglo, a $2.8 million possible cure for the rare blood disorder known as beta thalassaemia. It generally eliminates patients’ need for blood transfusions. The company is so sure it works that it will refund 80 percent of the cost of the therapy if patients need blood transfusions related to that condition within five years of being treated with Zynteglo.
In his February 2023 State of the Union speech, President Biden proposed three pilot programs to reduce drug costs. One of them, the Cell and Gene Therapy Access Model calls on the federal Centers for Medicare & Medicaid Services to establish outcomes-based agreements with manufacturers for certain cell and gene therapies.
A mortgage-style payment system is another, albeit rare, approach. Amortized payments spread the cost of treatments over decades, and let people change employers without losing their healthcare benefits.
Only about 14 percent of all drugs that enter clinical trials are approved by the FDA. Pharma companies, therefore, have an exigent need to earn a profit.
The new payment models that are being discussed aren’t solutions to high prices, says Bill Kramer, senior advisor for health policy at Purchaser Business Group on Health (PBGH), a nonprofit that seeks to lower health care costs. He points out that innovative pricing models, although well-intended, may distract from the real problem of high prices. They are attempts to “soften the blow. The best thing would be to charge a reasonable price to begin with,” he says.
Instead, he proposes making better use of research on cost and clinical effectiveness. The Institute for Clinical and Economic Review (ICER) conducts such research in the U.S., determining whether the benefits of specific drugs justify their proposed prices. ICER is an independent non-profit research institute. Its reports typically assess the degrees of improvement new therapies offer and suggest prices that would reflect that. “Publicizing that data is very important,” Kramer says. “Their results aren’t used to the extent they could and should be.” Pharmaceutical companies tend to price their therapies higher than ICER’s recommendations.
Drug Development Costs Soar
Drug developers have long pointed to the onerous costs of drug development as a reason for high prices.
A 2020 study found the average cost to bring a drug to market exceeded $1.1 billion, while other studies have estimated overall costs as high as $2.6 billion. The development timeframe is about 10 years. That’s because modern therapeutics target precise mechanisms to create better outcomes, but also have high failure rates. Only about 14 percent of all drugs that enter clinical trials are approved by the FDA. Pharma companies, therefore, have an exigent need to earn a profit.
Skewed Incentives Increase Costs
Pricing isn’t solely at the discretion of pharma companies, though. “What patients end up paying has much more to do with their PBMs than the actual price of the drug,” Patricia Goldsmith, CEO, CancerCare, says. Transparency is vital.
PBMs control patients’ access to therapies at three levels, through price negotiations, pricing tiers and pharmacy management.
When negotiating with drug manufacturers, Goldsmith says, “PBMs exchange a preferred spot on a formulary (the insurer’s or healthcare provider’s list of acceptable drugs) for cash-base rebates.” Unfortunately, 25 percent of the time, those rebates are not passed to insurers, according to the PBGH report.
Then, PBMs use pricing tiers to steer patients and physicians to certain drugs. For example, Kramer says, “Sometimes PBMs put a high-cost brand name drug in a preferred tier and a lower-cost competitor in a less preferred, higher-cost tier.” As the PBGH report elaborates, “(PBMs) are incentivized to include the highest-priced drugs…since both manufacturing rebates, as well as the administrative fees they charge…are calculated as a percentage of the drug’s price.
Finally, by steering patients to certain pharmacies, PBMs coordinate patients’ access to treatments, control patients’ out-of-pocket costs and receive management fees from the pharmacies.
Therefore, Goldsmith says, “As long as formularies are based on profits to middlemen…Americans’ healthcare costs will continue to skyrocket.”
Transparency into drug pricing will help curb costs, as will new payment strategies. What will make the most impact, however, may well be the development of a new reimbursement system designed to handle dramatic, breakthrough drugs. As Kramer says, “We need a better system to identify drugs that offer dramatic improvements in clinical care.”