Novel Technologies Could Make Coronavirus Vaccines More Stable for Worldwide Shipping
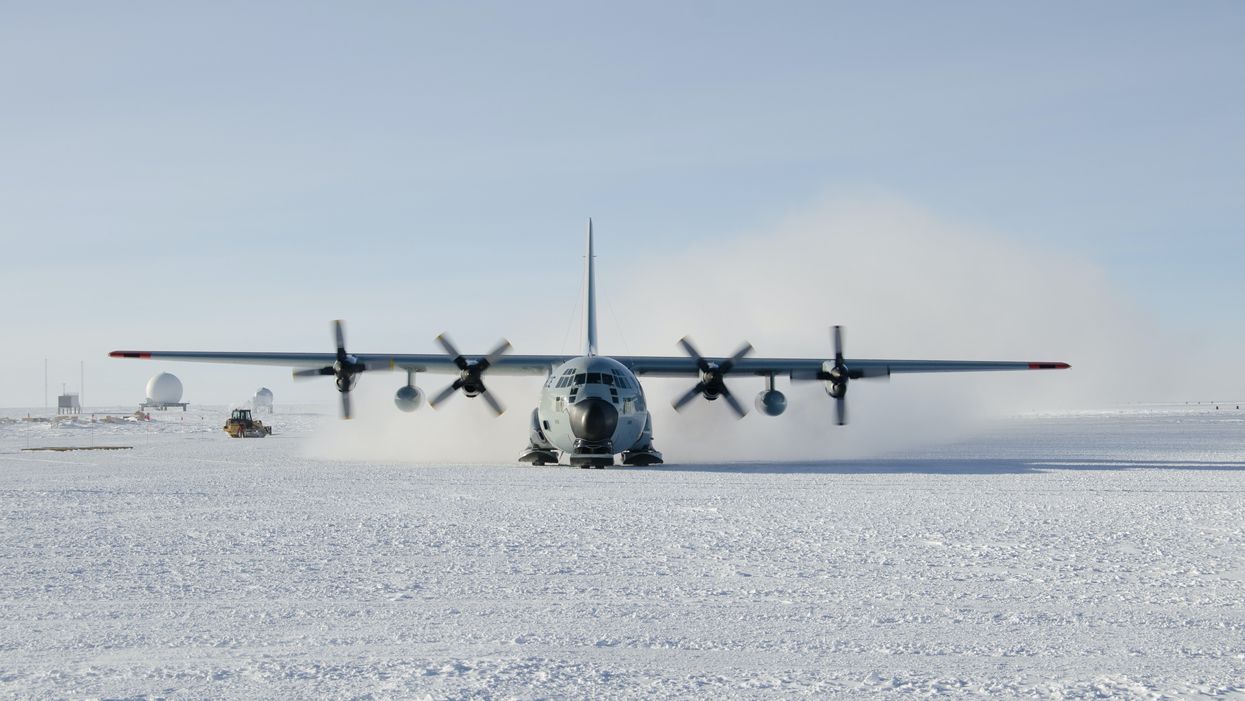
The vaccine from Pfizer will need to be stored at minus 70 degrees Celsius for worldwide distribution.
Ssendi Bosco has long known to fear the rainy season. As deputy health officer of Mubende District, a region in Central Uganda, she is only too aware of the threat that heavy storms can pose to her area's fragile healthcare facilities.
In early October, persistent rain overwhelmed the power generator that supplies electricity to most of the region, causing a blackout for three weeks. The result was that most of Mubende's vaccine supplies against diseases such as tuberculosis, diphtheria, and polio went to waste. "The vaccines need to be constantly refrigerated, so the generator failing means that most of them are now unusable," she says.
This week, the global fight against the coronavirus pandemic received a major boost when Pfizer and their German partner BioNTech released interim results showing that their vaccine has proved more than 90 percent effective at preventing participants in their clinical trial from getting COVID-19.
But while Pfizer has already signed deals to supply the vaccine to the U.S., U.K., Canada, Japan and the European Union, Mubende's recent plight provides an indication of the challenges that distributors will face when attempting to ship a coronavirus vaccine around the globe, particularly to low-income nations.
Experts have estimated that somewhere between 12 billion and 15 billion doses will be needed to immunize the world's population against COVID-19, a staggering scale, and one that has never been attempted before. "The logistics of distributing COVID-19 vaccines have been described as one of the biggest challenges in the history of mankind," says Göran Conradson, managing director of Swedish vaccine manufacturer Ziccum.
But even these estimates do not take into account the potential for vaccine spoilage. Every year, the World Health Organization estimates that over half of the world's vaccines end up being wasted. This happens because vaccines are fragile products. From the moment they are made, to the moment they are administered, they have to be kept within a tightly controlled temperature range. Throughout the entire supply chain – transportation to an airport, the flight to another country, unloaded, distribution via trucks to healthcare facilities, and storage – they must be refrigerated at all times. This is known as the cold chain, and one tiny slip along the way means the vaccines are ruined.
"It's a chain, and any chain is only as strong as its weakest link," says Asel Sartbaeva, a chemist working on vaccine technologies at the University of Bath in the U.K.
For COVID-19, the challenge is even greater because some of the leading vaccine candidates need to be kept at ultracold temperatures. Pfizer's vaccine, for example, must be kept at -70 degrees Celsius, the kind of freezer capabilities rarely found outsides of specialized laboratories. Transporting such a vaccine across North America and Europe will be difficult enough, but supplying it to some of the world's poorest nations in Asia, Africa and South America -- where only 10 percent of healthcare facilities have reliable electricity -- might appear virtually impossible.
But technology may be able to come to the rescue.
Making Vaccines Less Fragile
Just as the world's pharmaceutical companies have been racing against the clock to develop viable COVID-19 vaccine candidates, scientists around the globe have been hastily developing new technologies to try and make vaccines less fragile. Some approaches involve various chemicals that can be added to the vaccine to make them far more resilient to temperature fluctuations during transit, while others focus on insulated storage units that can maintain the vaccine at a certain temperature even if there is a power outage.
Some of these concepts have already been considered for several years, but before COVID-19 there was less of a commercial incentive to bring them to market. "We never felt that there is a need for an investment in this area," explains Sam Kosari, a pharmacist at the University of Canberra, who researches the vaccine cold chain. "Some technologies were developed then to assist with vaccine transport in Africa during Ebola, but since that outbreak was contained, there hasn't been any serious initiative or reward to develop this technology further."
In her laboratory at the University of Bath, Sartbaeva is using silica - the main constituent of sand – to encase the molecular components within a vaccine. Conventional vaccines typically contain protein targets from the virus, which the immune system learns to recognize. However, when they are exposed to temperature changes, these protein structures degrade, and lose their shape, making the vaccine useless. Sartbaeva compares this to how an egg changes its shape and consistency when it is boiled.
When silica is added to a vaccine, it molds to each protein, forming little protective cages around them, and thus preventing them from being affected by temperature changes. "The whole idea is that if we can create a shell around each protein, we can protect it from physically unravelling which is what causes the deactivation of the vaccine," she says.
Other scientists are exploring similar methods of making vaccines more resilient. Researchers at the Jenner Institute at the University of Oxford recently conducted a clinical trial in which they added carbohydrates to a dengue vaccine, to assess whether it became easier to transport.
Both research groups are now hoping to collaborate with the COVID-19 vaccine candidates being developed by AstraZeneca and Imperial College, assuming they become available in 2021.
"It's good we're all working on this big problem, as different methods could work better for different types of COVID-19 vaccines," says Sartbaeva. "I think it will be needed."
Next-Generation Vaccine Technology
While these different technologies could be utilized to try and protect the first wave of COVID-19 vaccines, efforts are also underway to develop completely new methods of vaccination. Much of this research is still in its earliest stages, but it could yield a second generation of COVID-19 vaccine candidates in 2022 and beyond.
"After the first round of mass vaccination, we could well observe regional outbreaks of the disease appearing from time to time in the coming years," says Kosari. "This is the time where new types of vaccines could be helpful."
One novel method being explored by Ziccum and others is dry powder vaccines. The idea is to spray dry the final vaccine into a powder form, where it is more easily preserved and does not require any special cooling while being transported or stored. People then receive the vaccine by inhaling it, rather than having it injected into their bloodstream.
Conradson explains that the concept of dry powder vaccines works on the same principle as dried food products. Because there is no water involved, the vaccine's components are far less affected by temperature changes. "It is actually the water that leads to the destruction of potency of a vaccine when it gets heated," he says. "We're looking to develop a dry powder vaccine for COVID-19 but this will be a second-generation vaccine. At the moment there are more than 200 first-generation candidates, all of which are using conventional technologies due to the timeframe pressures, which I think was the correct decision."
Dry powder COVID-19 vaccines could also be combined with microneedle patches, to allow people to self-administer the vaccine themselves in their own home. Microneedles are miniature needles – measured in millionths of a meter – which are designed to deliver medicines through the skin with minimal pain. So far, they have been used mainly in cosmetic products, but many scientists are working to use them to deliver drugs or vaccines.
At Georgia Institute of Technology in Atlanta, Mark Prausnitz is leading a couple of projects looking at incorporating COVID-19 vaccines into microneedle patches with the hope of running some early-stage clinical trials over the next couple of years. "The advantage is that they maintain the vaccine in a stable, dry state until it dissolves in the skin," he explains.
Prausnitz and others believe that once the first generation of COVID-19 vaccines become available, biotech and pharmaceutical companies will show more interest in adapting their products so they can be used in a dried form or with a microneedle patch. "There is so much pressure to get the COVID vaccine out that right now, vaccine developers are not interested in incorporating a novel delivery method," he says. "That will have to come later, once the pressure is lessened."
The Struggle of Low-Income Nations
For low-income nations, time will only tell whether technological advancements can enable them to access the first wave of licensed COVID-19 vaccines. But reports already suggest that they are in danger of becoming an afterthought in the race to procure vaccine supplies.
While initiatives such as COVAX are attempting to make sure that vaccine access is equitable, high and middle-income countries have already inked deals to secure 3.8 billion doses, with options for another 5 billion. One particularly sobering study by the Duke Global Health Innovation Center has suggested that such hoarding means many low-income nations may not receive a vaccine until 2024.
For Bosco and the residents of Mubende District in Uganda, all they can do is wait. In the meantime, there is a more pressing problem: fixing their generators. "We hope that we can receive a vaccine," she says. "But the biggest problem will be finding ways to safely store it. Right now we cannot keep any medicines or vaccines in the conditions they need, because we don't have the funds to repair our power generators."
Doctors worry that fungal pathogens may cause the next pandemic.
Bacterial antibiotic resistance has been a concern in the medical field for several years. Now a new, similar threat is arising: drug-resistant fungal infections. The Centers for Disease Control and Prevention considers antifungal and antimicrobial resistance to be among the world’s greatest public health challenges.
One particular type of fungal infection caused by Candida auris is escalating rapidly throughout the world. And to make matters worse, C. auris is becoming increasingly resistant to current antifungal medications, which means that if you develop a C. auris infection, the drugs your doctor prescribes may not work. “We’re effectively out of medicines,” says Thomas Walsh, founding director of the Center for Innovative Therapeutics and Diagnostics, a translational research center dedicated to solving the antimicrobial resistance problem. Walsh spoke about the challenges at a Demy-Colton Virtual Salon, one in a series of interactive discussions among life science thought leaders.
Although C. auris typically doesn’t sicken healthy people, it afflicts immunocompromised hospital patients and may cause severe infections that can lead to sepsis, a life-threatening condition in which the overwhelmed immune system begins to attack the body’s own organs. Between 30 and 60 percent of patients who contract a C. auris infection die from it, according to the CDC. People who are undergoing stem cell transplants, have catheters or have taken antifungal or antibiotic medicines are at highest risk. “We’re coming to a perfect storm of increasing resistance rates, increasing numbers of immunosuppressed patients worldwide and a bug that is adapting to higher temperatures as the climate changes,” says Prabhavathi Fernandes, chair of the National BioDefense Science Board.
Most Candida species aren’t well-adapted to our body temperatures so they aren’t a threat. C. auris, however, thrives at human body temperatures.
Although medical professionals aren’t concerned at this point about C. auris evolving to affect healthy people, they worry that its presence in hospitals can turn routine surgeries into life-threatening calamities. “It’s coming,” says Fernandes. “It’s just a matter of time.”
An emerging global threat
“Fungi are found in the environment,” explains Fernandes, so Candida spores can easily wind up on people’s skin. In hospitals, they can be transferred from contact with healthcare workers or contaminated surfaces. Most Candida species aren’t well-adapted to our body temperatures so they aren’t a threat. C. auris, however, thrives at human body temperatures. It can enter the body during medical treatments that break the skin—and cause an infection. Overall, fungal infections cost some $48 billion in the U.S. each year. And infection rates are increasing because, in an ironic twist, advanced medical therapies are enabling severely ill patients to live longer and, therefore, be exposed to this pathogen.
The first-ever case of a C. auris infection was reported in Japan in 2009, although an analysis of Candida samples dated the earliest strain to a 1996 sample from South Korea. Since then, five separate varieties – called clades, which are similar to strains among bacteria – developed independently in different geographies: South Asia, East Asia, South Africa, South America and, recently, Iran. So far, C. auris infections have been reported in 35 countries.
In the U.S., the first infection was reported in 2016, and the CDC started tracking it nationally two years later. During that time, 5,654 cases have been reported to the CDC, which only tracks U.S. data.
What’s more notable than the number of cases is their rate of increase. In 2016, new cases increased by 175 percent and, on average, they have approximately doubled every year. From 2016 through 2022, the number of infections jumped from 63 to 2,377, a roughly 37-fold increase.
“This reminds me of what we saw with epidemics from 2013 through 2020… with Ebola, Zika and the COVID-19 pandemic,” says Robin Robinson, CEO of Spriovas and founding director of the Biomedical Advanced Research and Development Authority (BARDA), which is part of the U.S. Department of Health and Human Services. These epidemics started with a hockey stick trajectory, Robinson says—a gradual growth leading to a sharp spike, just like the shape of a hockey stick.
Another challenge is that right now medics don’t have rapid diagnostic tests for fungal infections. Currently, patients are often misdiagnosed because C. auris resembles several other easily treated fungi. Or they are diagnosed long after the infection begins and is harder to treat.
The problem is that existing diagnostics tests can only identify C. auris once it reaches the bloodstream. Yet, because this pathogen infects bodily tissues first, it should be possible to catch it much earlier before it becomes life-threatening. “We have to diagnose it before it reaches the bloodstream,” Walsh says.
The most alarming fact is that some Candida infections no longer respond to standard therapeutics.
“We need to focus on rapid diagnostic tests that do not rely on a positive blood culture,” says John Sperzel, president and CEO of T2 Biosystems, a company specializing in diagnostics solutions. Blood cultures typically take two to three days for the concentration of Candida to become large enough to detect. The company’s novel test detects about 90 percent of Candida species within three to five hours—thanks to its ability to spot minute quantities of the pathogen in blood samples instead of waiting for them to incubate and proliferate.
Unlike other Candida species C. auris thrives at human body temperatures
Adobe Stock
Tackling the resistance challenge
The most alarming fact is that some Candida infections no longer respond to standard therapeutics. The number of cases that stopped responding to echinocandin, the first-line therapy for most Candida infections, tripled in 2020, according to a study by the CDC.
Now, each of the first four clades shows varying levels of resistance to all three commonly prescribed classes of antifungal medications, such as azoles, echinocandins, and polyenes. For example, 97 percent of infections from C. auris Clade I are resistant to fluconazole, 54 percent to voriconazole and 30 percent of amphotericin. Nearly half are resistant to multiple antifungal drugs. Even with Clade II fungi, which has the least resistance of all the clades, 11 to 14 percent have become resistant to fluconazole.
Anti-fungal therapies typically target specific chemical compounds present on fungi’s cell membranes, but not on human cells—otherwise the medicine would cause damage to our own tissues. Fluconazole and other azole antifungals target a compound called ergosterol, preventing the fungal cells from replicating. Over the years, however, C. auris evolved to resist it, so existing fungal medications don’t work as well anymore.
A newer class of drugs called echinocandins targets a different part of the fungal cell. “The echinocandins – like caspofungin – inhibit (a part of the fungi) involved in making glucan, which is an essential component of the fungal cell wall and is not found in human cells,” Fernandes says. New antifungal treatments are needed, she adds, but there are only a few magic bullets that will hit just the fungus and not the human cells.
Research to fight infections also has been challenged by a lack of government support. That is changing now that BARDA is requesting proposals to develop novel antifungals. “The scope includes C. auris, as well as antifungals following a radiological/nuclear emergency, says BARDA spokesperson Elleen Kane.
The remaining challenge is the number of patients available to participate in clinical trials. Large numbers are needed, but the available patients are quite sick and often die before trials can be completed. Consequently, few biopharmaceutical companies are developing new treatments for C. auris.
ClinicalTrials.gov reports only two drugs in development for invasive C. auris infections—those than can spread throughout the body rather than localize in one particular area, like throat or vaginal infections: ibrexafungerp by Scynexis, Inc., fosmanogepix, by Pfizer.
Scynexis’ ibrexafungerp appears active against C. auris and other emerging, drug-resistant pathogens. The FDA recently approved it as a therapy for vaginal yeast infections and it is undergoing Phase III clinical trials against invasive candidiasis in an attempt to keep the infection from spreading.
“Ibreafungerp is structurally different from other echinocandins,” Fernandes says, because it targets a different part of the fungus. “We’re lucky it has activity against C. auris.”
Pfizer’s fosmanogepix is in Phase II clinical trials for patients with invasive fungal infections caused by multiple Candida species. Results are showing significantly better survival rates for people taking fosmanogepix.
Although C. auris does pose a serious threat to healthcare worldwide, scientists try to stay optimistic—because they recognized the problem early enough, they might have solutions in place before the perfect storm hits. “There is a bit of hope,” says Robinson. “BARDA has finally been able to fund the development of new antifungal agents and, hopefully, this year we can get several new classes of antifungals into development.”
New elevators could lift up our access to space
A space elevator would be cheaper and cleaner than using rockets
Story by Big Think
When people first started exploring space in the 1960s, it cost upwards of $80,000 (adjusted for inflation) to put a single pound of payload into low-Earth orbit.
A major reason for this high cost was the need to build a new, expensive rocket for every launch. That really started to change when SpaceX began making cheap, reusable rockets, and today, the company is ferrying customer payloads to LEO at a price of just $1,300 per pound.
This is making space accessible to scientists, startups, and tourists who never could have afforded it previously, but the cheapest way to reach orbit might not be a rocket at all — it could be an elevator.
The space elevator
The seeds for a space elevator were first planted by Russian scientist Konstantin Tsiolkovsky in 1895, who, after visiting the 1,000-foot (305 m) Eiffel Tower, published a paper theorizing about the construction of a structure 22,000 miles (35,400 km) high.
This would provide access to geostationary orbit, an altitude where objects appear to remain fixed above Earth’s surface, but Tsiolkovsky conceded that no material could support the weight of such a tower.
We could then send electrically powered “climber” vehicles up and down the tether to deliver payloads to any Earth orbit.
In 1959, soon after Sputnik, Russian engineer Yuri N. Artsutanov proposed a way around this issue: instead of building a space elevator from the ground up, start at the top. More specifically, he suggested placing a satellite in geostationary orbit and dropping a tether from it down to Earth’s equator. As the tether descended, the satellite would ascend. Once attached to Earth’s surface, the tether would be kept taut, thanks to a combination of gravitational and centrifugal forces.
We could then send electrically powered “climber” vehicles up and down the tether to deliver payloads to any Earth orbit. According to physicist Bradley Edwards, who researched the concept for NASA about 20 years ago, it’d cost $10 billion and take 15 years to build a space elevator, but once operational, the cost of sending a payload to any Earth orbit could be as low as $100 per pound.
“Once you reduce the cost to almost a Fed-Ex kind of level, it opens the doors to lots of people, lots of countries, and lots of companies to get involved in space,” Edwards told Space.com in 2005.
In addition to the economic advantages, a space elevator would also be cleaner than using rockets — there’d be no burning of fuel, no harmful greenhouse emissions — and the new transport system wouldn’t contribute to the problem of space junk to the same degree that expendable rockets do.
So, why don’t we have one yet?
Tether troubles
Edwards wrote in his report for NASA that all of the technology needed to build a space elevator already existed except the material needed to build the tether, which needs to be light but also strong enough to withstand all the huge forces acting upon it.
The good news, according to the report, was that the perfect material — ultra-strong, ultra-tiny “nanotubes” of carbon — would be available in just two years.
“[S]teel is not strong enough, neither is Kevlar, carbon fiber, spider silk, or any other material other than carbon nanotubes,” wrote Edwards. “Fortunately for us, carbon nanotube research is extremely hot right now, and it is progressing quickly to commercial production.”Unfortunately, he misjudged how hard it would be to synthesize carbon nanotubes — to date, no one has been able to grow one longer than 21 inches (53 cm).
Further research into the material revealed that it tends to fray under extreme stress, too, meaning even if we could manufacture carbon nanotubes at the lengths needed, they’d be at risk of snapping, not only destroying the space elevator, but threatening lives on Earth.
Looking ahead
Carbon nanotubes might have been the early frontrunner as the tether material for space elevators, but there are other options, including graphene, an essentially two-dimensional form of carbon that is already easier to scale up than nanotubes (though still not easy).
Contrary to Edwards’ report, Johns Hopkins University researchers Sean Sun and Dan Popescu say Kevlar fibers could work — we would just need to constantly repair the tether, the same way the human body constantly repairs its tendons.
“Using sensors and artificially intelligent software, it would be possible to model the whole tether mathematically so as to predict when, where, and how the fibers would break,” the researchers wrote in Aeon in 2018.
“When they did, speedy robotic climbers patrolling up and down the tether would replace them, adjusting the rate of maintenance and repair as needed — mimicking the sensitivity of biological processes,” they continued.Astronomers from the University of Cambridge and Columbia University also think Kevlar could work for a space elevator — if we built it from the moon, rather than Earth.
They call their concept the Spaceline, and the idea is that a tether attached to the moon’s surface could extend toward Earth’s geostationary orbit, held taut by the pull of our planet’s gravity. We could then use rockets to deliver payloads — and potentially people — to solar-powered climber robots positioned at the end of this 200,000+ mile long tether. The bots could then travel up the line to the moon’s surface.
This wouldn’t eliminate the need for rockets to get into Earth’s orbit, but it would be a cheaper way to get to the moon. The forces acting on a lunar space elevator wouldn’t be as strong as one extending from Earth’s surface, either, according to the researchers, opening up more options for tether materials.
“[T]he necessary strength of the material is much lower than an Earth-based elevator — and thus it could be built from fibers that are already mass-produced … and relatively affordable,” they wrote in a paper shared on the preprint server arXiv.
After riding up the Earth-based space elevator, a capsule would fly to a space station attached to the tether of the moon-based one.
Electrically powered climber capsules could go up down the tether to deliver payloads to any Earth orbit.
Adobe Stock
Some Chinese researchers, meanwhile, aren’t giving up on the idea of using carbon nanotubes for a space elevator — in 2018, a team from Tsinghua University revealed that they’d developed nanotubes that they say are strong enough for a tether.
The researchers are still working on the issue of scaling up production, but in 2021, state-owned news outlet Xinhua released a video depicting an in-development concept, called “Sky Ladder,” that would consist of space elevators above Earth and the moon.
After riding up the Earth-based space elevator, a capsule would fly to a space station attached to the tether of the moon-based one. If the project could be pulled off — a huge if — China predicts Sky Ladder could cut the cost of sending people and goods to the moon by 96 percent.
The bottom line
In the 120 years since Tsiolkovsky looked at the Eiffel Tower and thought way bigger, tremendous progress has been made developing materials with the properties needed for a space elevator. At this point, it seems likely we could one day have a material that can be manufactured at the scale needed for a tether — but by the time that happens, the need for a space elevator may have evaporated.
Several aerospace companies are making progress with their own reusable rockets, and as those join the market with SpaceX, competition could cause launch prices to fall further.
California startup SpinLaunch, meanwhile, is developing a massive centrifuge to fling payloads into space, where much smaller rockets can propel them into orbit. If the company succeeds (another one of those big ifs), it says the system would slash the amount of fuel needed to reach orbit by 70 percent.
Even if SpinLaunch doesn’t get off the ground, several groups are developing environmentally friendly rocket fuels that produce far fewer (or no) harmful emissions. More work is needed to efficiently scale up their production, but overcoming that hurdle will likely be far easier than building a 22,000-mile (35,400-km) elevator to space.
This article originally appeared on Big Think, home of the brightest minds and biggest ideas of all time.