Sustainable Urban Farming Has a Rising Hot Star: Bugs
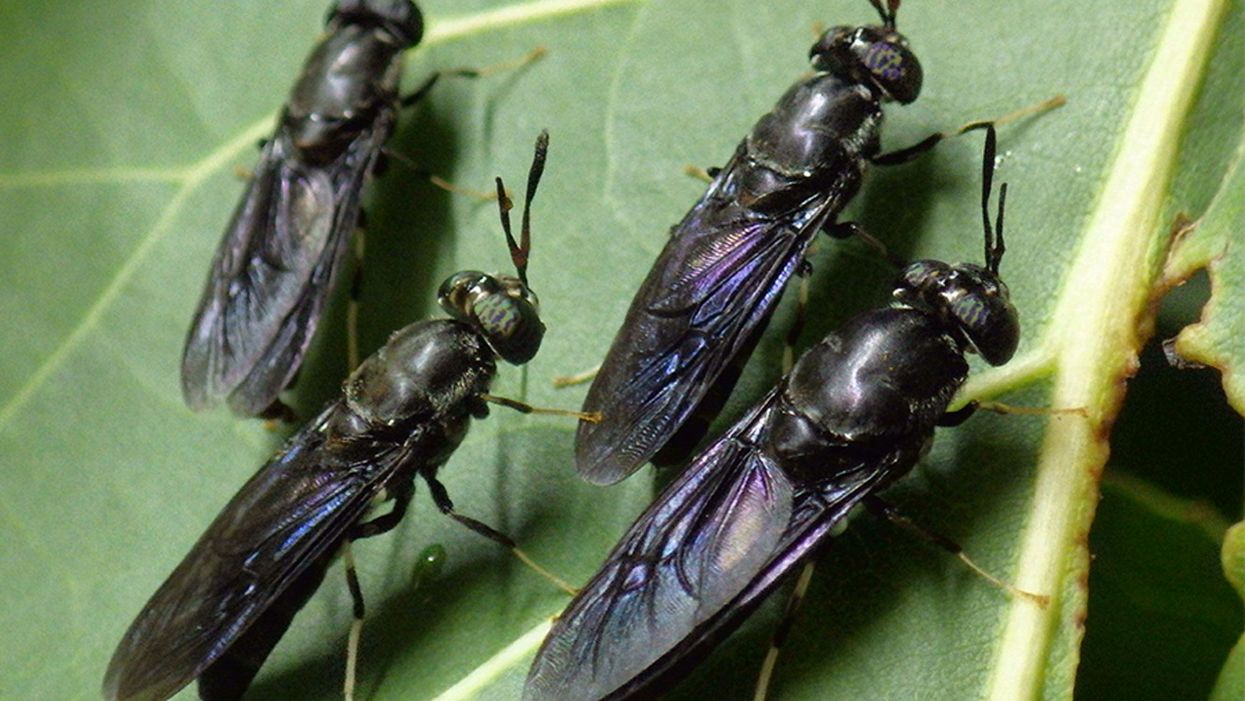
The larvae of adult black soldier flies can turn food waste into sustainable protein with minimal methane gas emissions.
In Sydney, Australia, in the basement of an inner-city high-rise, lives a mass of unexpected inhabitants: millions of maggots. The insects are far from unwelcome. They are there to feast on the food waste generated by the building's human residents.
Goterra, the start-up that installed the maggots in the building in December, belongs to the rapidly expanding insect agriculture industry, which is experiencing a surge of investment worldwide.
The maggots – the larvae of the black soldier fly – are voracious, unfussy eaters. As adult flies, they don't eat, so the young fatten up swiftly on whatever they can get. Goterra's basement colony can munch through 5 metric tons of waste in a day.
"Maggots are nature's cleaners," says Bob Gordon, Head of Growth at Goterra. "They're a great tool to manage waste streams."
Their capacity to consume presents a neat response to the problem of food waste, which contributes up to 8% of global greenhouse gas emissions each year as it rots in landfill.
"The maggots eat the food fairly fresh," Gordon says. "So, there's minimal degradation and you don't get those methane emissions."
Alongside their ability to devour waste, the soldier fly larvae hold further agricultural promise: they yield an incredibly efficient protein. After the maggots have binged for about 12 days, Goterra harvests and processes them into a protein-rich livestock feed. Their excrement, known as frass, is also collected and turned into soil conditioner.
"We are producing protein in a basement," says Gordon. "It's urban farming – really sustainable, urban farming."
Goterra's module in the basement at Barangaroo, Sydney.
Supplied by Goterra
Goterra's founder Olympia Yarger started producing the insects in "buckets in her backyard" in 2016. Today, Goterra has a large-scale processing plant and has developed proprietary modules – in shipping containers – that use robotics to manage the larvae.
The modules have been installed on site at municipal buildings, hospitals, supermarkets, several McDonald's restaurants, and a range of smaller enterprises in Australia. Users pay a subscription fee and simply pour in the waste; Goterra visits once a fortnight to harvest the bugs.
Insect agriculture is well established outside of the West, and the practice is gaining traction around the world. China has mega-facilities that can process hundreds of tons of waste in a day. In Kenya, a program recently trained 2000 farmers in soldier fly farming to boost their economic security. French biotech company InnovaFeed, in partnership with US agricultural heavyweight ADM, plans to build "the world's largest insect protein facility" in Illinois this year.
"The [maggots] are science fiction on earth. Watching them work is awe-inspiring."
But the concept is still not to everyone's taste.
"This is still a topic that I say is a bit like black liquorice – people tend to either really like it or really don't," says Wendy Lu McGill, Communications Director at the North American Coalition of Insect Agriculture (NACIA).
Formed in 2016, NACIA now has over 100 members – including researchers and commercial producers of black soldier flies, meal worms and crickets.
McGill says there have been a few iterations of insect agriculture in the US – beginning with worms produced for bait after World War II then shifting to food for exotic pets. The current focus – "insects as food and feed" – took root about a decade ago, with the establishment of the first commercial farms for this purpose.
"We're starting to see more expansion in the U.S. and a lot of the larger investments have been for black soldier fly producers," McGill says. "They tend to have larger facilities and the animal feed market they're looking at is potentially quite large."
InnovaFeed's Illinois facility is set to produce 60,000 metric tons of animal feed protein per year.
"They'll be trying to employ many different circular principles," McGill says of the project. "For example, the heat from the feed factory – the excess heat that would normally just be vented – will be used to heat the other side that's raising the black soldier fly."
Although commercial applications have started to flourish recently, scientific knowledge of the black soldier fly's potential has existed for decades.
Dr. Jeffery Tomberlin, an entomologist at Texas A&M University, has been studying the insect for over 20 years, contributing to key technologies used in the industry. He also founded Evo, a black soldier fly company in Texas, which feeds its larvae the waste from a local bakery and distillery.
"They are science fiction on earth," he says of the maggots. "Watching them work is awe-inspiring."
Tomberlin says fly farms can work effectively at different scales, and present possibilities for non-Western countries to shift towards "commodity independence."
"You don't have to have millions of dollars invested to be successful in producing this insect," he says. "[A farm] can be as simple as an open barn along the equator to a 30,000 square-foot indoor facility in the Netherlands."
As the world's population balloons, food insecurity is an increasing concern. By 2050, the UN predicts that to feed our projected population we will need to ramp up food production by at least 60%. Insect agriculture, which uses very little land and water compared to traditional livestock farming, could play a key role.
Insects may become more common human food, but the current commercial focus is animal feed. Aquaculture is a key market, with insects presenting an alternative to fish meal derived from over-exploited stocks. Insect meal is also increasingly popular in pet food, particularly in Europe.
While recent investment has been strong – NACIA says 2020 was the best year yet – reaching a scale that can match existing agricultural industries and providing a competitive price point are still hurdles for insect agriculture.
But COVID-19 has strengthened the argument for new agricultural approaches, such as the decentralized, indoor systems and circular principles employed by insect farms.
"This has given the world a preview – which no one wanted – of [future] supply chain disruptions," says McGill.
As the industry works to meet demand, Tomberlin predicts diversification and product innovation: "I think food science is going to play a big part in that. They can take an insect and create ice cream." (Dried soldier fly larvae "taste kind of like popcorn," if you were wondering.)
Tomberlin says the insects could even become an interplanetary protein source: "I do believe in that. I mean, if we're going to colonize other planets, we need to be sustainable."
But he issues a word of caution about the industry growing too big, too fast: "I think we as an industry need to be very careful of how we harness and apply [our knowledge]. The black soldier fly is considered the crown jewel today, but if it's mismanaged, it can be relegated back to a past."
Goterra's Gordon also warns against rushing into mass production: "If you're just replacing big intensive animal agriculture with big intensive animal agriculture with more efficient animals, then what's the change you're really effecting?"
But he expects the industry will continue its rise though the next decade, and Goterra – fuelled by recent $8 million Series A funding – plans to expand internationally this year.
"Within 10 years' time, I would like to see the vast majority of our unavoidable food waste being used to produce maggots to go into a protein application," Gordon says.
"There's no lack of demand. And there's no lack of food waste."
Dr. Steffanie Strathdee, an infectious disease epidemiologist, saved her husband Tom from a superbug infection by mobilizing scientists around the world to help him access experimental phage therapy.
[Editor's Note: This is the fifth episode in our Moonshot series, which explores cutting-edge scientific developments that stand to fundamentally transform our world.]
Kira Peikoff was the editor-in-chief of Leaps.org from 2017 to 2021. As a journalist, her work has appeared in The New York Times, Newsweek, Nautilus, Popular Mechanics, The New York Academy of Sciences, and other outlets. She is also the author of four suspense novels that explore controversial issues arising from scientific innovation: Living Proof, No Time to Die, Die Again Tomorrow, and Mother Knows Best. Peikoff holds a B.A. in Journalism from New York University and an M.S. in Bioethics from Columbia University. She lives in New Jersey with her husband and two young sons. Follow her on Twitter @KiraPeikoff.
The latest tools, like whole genome sequencing, are allowing food outbreaks to be identified and stopped more quickly.
With the pandemic at the forefront of everyone's minds, many people have wondered if food could be a source of coronavirus transmission. Luckily, that "seems unlikely," according to the CDC, but foodborne illnesses do still sicken a whopping 48 million people per year.
Whole genome sequencing is like "going from an eight-bit image—maybe like what you would see in Minecraft—to a high definition image."
In normal times, when there isn't a historic global health crisis infecting millions and affecting the lives of billions, foodborne outbreaks are real and frightening, potentially deadly, and can cause widespread fear of particular foods. Think of Romaine lettuce spreading E. coli last year— an outbreak that infected more than 500 people and killed eight—or peanut butter spreading salmonella in 2008, which infected 167 people.
The technologies available to detect and prevent the next foodborne disease outbreak have improved greatly over the past 30-plus years, particularly during the past decade, and better, more nimble technologies are being developed, according to experts in government, academia, and private industry. The key to advancing detection of harmful foodborne pathogens, they say, is increasing speed and portability of detection, and the precision of that detection.
Getting to Rapid Results
Researchers at Purdue University have recently developed a lateral flow assay that, with the help of a laser, can detect toxins and pathogenic E. coli. Lateral flow assays are cheap and easy to use; a good example is a home pregnancy test. You place a liquid or liquefied sample on a piece of paper designed to detect a single substance and soon after you get the results in the form of a colored line: yes or no.
"They're a great portable tool for us for food contaminant detection," says Carmen Gondhalekar, a fifth-year biomedical engineering graduate student at Purdue. "But one of the areas where paper-based lateral flow assays could use improvement is in multiplexing capability and their sensitivity."
J. Paul Robinson, a professor in Purdue's Colleges of Veterinary Medicine and Engineering, and Gondhalekar's advisor, agrees. "One of the fundamental problems that we have in detection is that it is hard to identify pathogens in complex samples," he says.
When it comes to foodborne disease outbreaks, you don't always know what substance you're looking for, so an assay made to detect only a single substance isn't always effective. The goal of the project at Purdue is to make assays that can detect multiple substances at once.
These assays would be more complex than a pregnancy test. As detailed in Gondhalekar's recent paper, a laser pulse helps create a spectral signal from the sample on the assay paper, and the spectral signal is then used to determine if any unique wavelengths associated with one of several toxins or pathogens are present in the sample. Though the handheld technology has yet to be built, the idea is that the results would be given on the spot. So someone in the field trying to track the source of a Salmonella infection could, for instance, put a suspected lettuce sample on the assay and see if it has the pathogen on it.
"What our technology is designed to do is to give you a rapid assessment of the sample," says Robinson. "The goal here is speed."
Seeing the Pathogen in "High-Def"
"One in six Americans will get a foodborne illness every year," according to Dr. Heather Carleton, a microbiologist at the Centers for Disease Control and Prevention's Enteric Diseases Laboratory Branch. But not every foodborne outbreak makes the news. In 2017 alone, the CDC monitored between 18 and 37 foodborne poison clusters per week and investigated 200 multi-state clusters. Hardboiled eggs, ground beef, chopped salad kits, raw oysters, frozen tuna, and pre-cut melon are just a taste of the foods that were investigated last year for different strains of listeria, salmonella, and E. coli.
At the heart of the CDC investigations is PulseNet, a national network of laboratories that uses DNA fingerprinting to detect outbreaks at local and regional levels. This is how it works: When a patient gets sick—with symptoms like vomiting and fever, for instance—they will go to a hospital or clinic for treatment. Since we're talking about foodborne illnesses, a clinician will likely take a stool sample from the patient and send it off to a laboratory to see if there is a foodborne pathogen, like salmonella, E. Coli, or another one. If it does contain a potentially harmful pathogen, then a bacterial isolate of that identified sample is sent to a regional public health lab so that whole genome sequencing can be performed.
Whole genome sequencing can differentiate "virtually all" strains of foodborne pathogens, no matter the species, according to the FDA.
Whole genome sequencing is a method for reading the entire genome of a bacterial isolate (or from any organism, for that matter). Instead of working with a couple dozen data points, now you're working with millions of base pairs. Carleton likes to describe it as "going from an eight-bit image—maybe like what you would see in Minecraft—to a high definition image," she says. "It's really an evolution of how we detect foodborne illnesses and identify outbreaks."
If the bacterial isolate matches another in the CDC's database, this means there could be a potential outbreak and an investigation may be started, with the goal of tracking the pathogen to its source.
Whole genome sequencing has been a relatively recent shift in foodborne disease detection. For more than 20 years, the standard technique for analyzing pathogens in foodborne disease outbreaks was pulsed-field gel electrophoresis. This method creates a DNA fingerprint for each sample in the form of a pattern of about 15-30 "bands," with each band representing a piece of DNA. Researchers like Carleton can use this fingerprint to see if two samples are from the same bacteria. The problem is that 15-30 bands are not enough to differentiate all isolates. Some isolates whose bands look very similar may actually come from different sources and some whose bands look different may be from the same source. But if you can see the entire DNA fingerprint, then you don't have that issue. That's where whole genome sequencing comes in.
Although the PulseNet team had piloted whole genome sequencing as early as 2013, it wasn't until July of last year that the transition to using whole genome sequencing for all pathogens was complete. Though whole genome sequencing requires far more computing power to generate, analyze, and compare those millions of data points, the payoff is huge.
Stopping Outbreaks Sooner
The U.S. Food and Drug Administration (FDA) acquired their first whole genome sequencers in 2008, according to Dr. Eric Brown, the Director of the Division of Microbiology in the FDA's Office of Regulatory Science. Since then, through their GenomeTrakr program, a network of more than 60 domestic and international labs, the FDA has sequenced and publicly shared more than 400,000 isolates. "The impact of what whole genome sequencing could do to resolve a foodborne outbreak event was no less impactful than when NASA turned on the Hubble Telescope for the first time," says Brown.
Whole genome sequencing has helped identify strains of Salmonella that prior methods were unable to differentiate. In fact, whole genome sequencing can differentiate "virtually all" strains of foodborne pathogens, no matter the species, according to the FDA. This means it takes fewer clinical cases—fewer sick people—to detect and end an outbreak.
And perhaps the largest benefit of whole genome sequencing is that these detailed sequences—the millions of base pairs—can imply geographic location. The genomic information of bacterial strains can be different depending on the area of the country, helping these public health agencies eventually track the source of outbreaks—a restaurant, a farm, a food-processing center.
Coming Soon: "Lab in a Backpack"
Now that whole genome sequencing has become the go-to technology of choice for analyzing foodborne pathogens, the next step is making the process nimbler and more portable. Putting "the lab in a backpack," as Brown says.
The CDC's Carleton agrees. "Right now, the sequencer we use is a fairly big box that weighs about 60 pounds," she says. "We can't take it into the field."
A company called Oxford Nanopore Technologies is developing handheld sequencers. Their devices are meant to "enable the sequencing of anything by anyone anywhere," according to Dan Turner, the VP of Applications at Oxford Nanopore.
"The sooner that we can see linkages…the sooner the FDA gets in action to mitigate the problem and put in some kind of preventative control."
"Right now, sequencing is very much something that is done by people in white coats in laboratories that are set up for that purpose," says Turner. Oxford Nanopore would like to create a new, democratized paradigm.
The FDA is currently testing these types of portable sequencers. "We're very excited about it. We've done some pilots, to be able to do that sequencing in the field. To actually do it at a pond, at a river, at a canal. To do it on site right there," says Brown. "This, of course, is huge because it means we can have real-time sequencing capability to stay in step with an actual laboratory investigation in the field."
"The timeliness of this information is critical," says Marc Allard, a senior biomedical research officer and Brown's colleague at the FDA. "The sooner that we can see linkages…the sooner the FDA gets in action to mitigate the problem and put in some kind of preventative control."
At the moment, the world is rightly focused on COVID-19. But as the danger of one virus subsides, it's only a matter of time before another pathogen strikes. Hopefully, with new and advancing technology like whole genome sequencing, we can stop the next deadly outbreak before it really gets going.