Who’s Responsible If a Scientist’s Work Is Used for Harm?
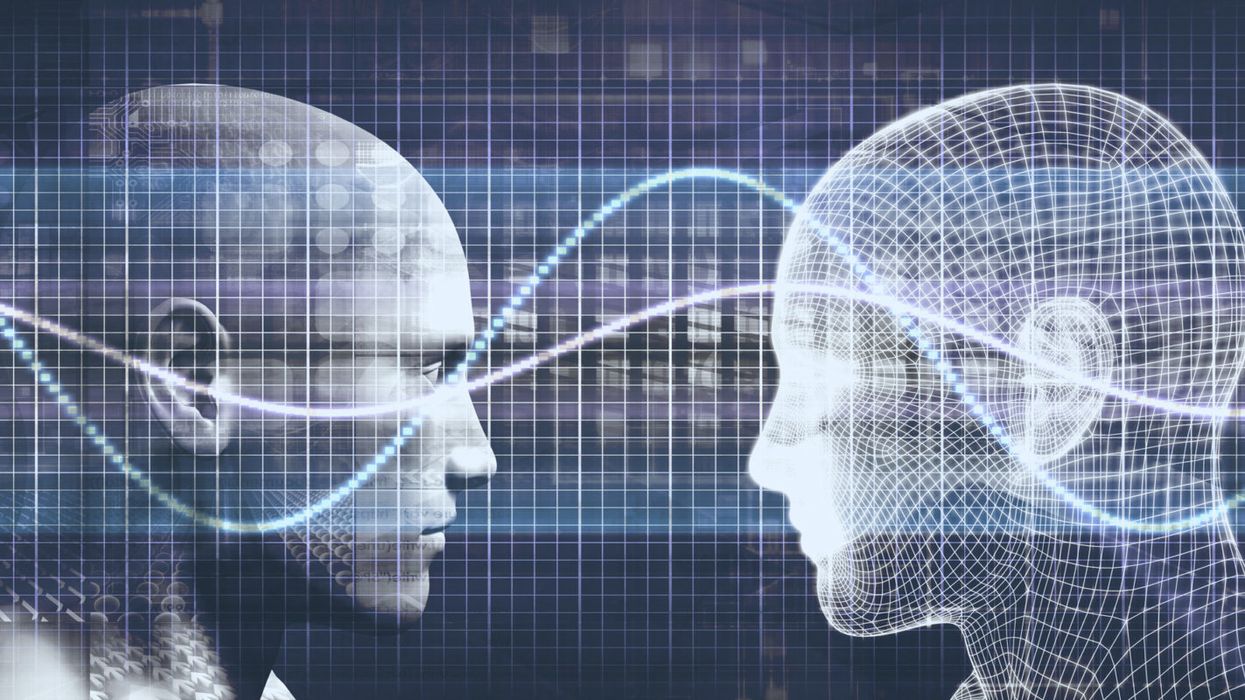
A face off in medical ethics.
Are scientists morally responsible for the uses of their work? To some extent, yes. Scientists are responsible for both the uses that they intend with their work and for some of the uses they don't intend. This is because scientists bear the same moral responsibilities that we all bear, and we are all responsible for the ends we intend to help bring about and for some (but not all) of those we don't.
To not think about plausible unintended effects is to be negligent -- and to recognize, but do nothing about, such effects is to be reckless.
It should be obvious that the intended outcomes of our work are within our sphere of moral responsibility. If a scientist intends to help alleviate hunger (by, for example, breeding new drought-resistant crop strains), and they succeed in that goal, they are morally responsible for that success, and we would praise them accordingly. If a scientist intends to produce a new weapon of mass destruction (by, for example, developing a lethal strain of a virus), and they are unfortunately successful, they are morally responsible for that as well, and we would blame them accordingly. Intention matters a great deal, and we are most praised or blamed for what we intend to accomplish with our work.
But we are responsible for more than just the intended outcomes of our choices. We are also responsible for unintended but readily foreseeable uses of our work. This is in part because we are all responsible for thinking not just about what we intend, but also what else might follow from our chosen course of action. In cases where severe and egregious harms are plausible, we should act in ways that strive to prevent such outcomes. To not think about plausible unintended effects is to be negligent -- and to recognize, but do nothing about, such effects is to be reckless. To be negligent or reckless is to be morally irresponsible, and thus blameworthy. Each of us should think beyond what we intend to do, reflecting carefully on what our course of action could entail, and adjusting our choices accordingly.
It is this area, of unintended but readily foreseeable (and plausible) impacts, that often creates the most difficulty for scientists. Many scientists can become so focused on their work (which is often demanding) and so focused on achieving their intended goals, that they fail to stop and think about other possible implications.
Debates over "dual-use" research exemplify these concerns, where harmful potential uses of research might mean the work should not be pursued, or the full publication of results should be curtailed. When researchers perform gain-of-function research, pushing viruses to become more transmissible or more deadly, it is clear how dangerous such work could be in the wrong hands. In these cases, it is not enough to simply claim that such uses were not intended and that it is someone else's job to ensure that the materials remain secure. We know securing infectious materials can be error-prone (recall events at the CDC and the FDA).
In some areas of research, scientists are already worrying about the unintended possible downsides of their work.
Further, securing viral strains does nothing to secure the knowledge that could allow for reproducing the viral strain (particularly when the methodologies and/or genetic sequences are published after the fact, as was the case for H5N1 and horsepox). It is, in fact, the researcher's moral responsibility to be concerned not just about the biosafety controls in their own labs, but also which projects should be pursued (Will the gain in knowledge be worth the possible downsides?) and which results should be published (Will a result make it easier for a malicious actor to deploy a new bioweapon?).
We have not yet had (to my knowledge) a use of gain-of-function research to harm people. If that does happen, those who actually released the virus on the public will be most blameworthy–-intentions do matter. But the scientists who developed the knowledge deployed by the malicious actors may also be held blameworthy, especially if the malicious use was easy to foresee, even if it was not pleasant to think about.
In some areas of research, scientists are already worrying about the unintended possible downsides of their work. Scientists investigating gene drives have thought beyond the immediate desired benefits of their work (e.g. reducing invasive species populations) and considered the possible spread of gene drives to untargeted populations. Modeling the impacts of such possibilities has led some researchers to pull back from particular deployment possibilities. It is precisely such thinking through both the intended and unintended possible outcomes that is needed for responsible work.
The world has gotten too small, too vulnerable for scientists to act as though they are not responsible for the uses of their work, intended or not. They must seek to ensure that, as the recent AAAS Statement on Scientific Freedom and Responsibility demands, their work is done "in the interest of humanity." This requires thinking beyond one's intentions, potentially drawing on the expertise of others, sometimes from other disciplines, to help explore implications. The need for such thinking does not guarantee good outcomes, but it will ensure that we are doing the best we can, and that is what being morally responsible is all about.
Fast for Longevity, with Less Hunger, with Dr. Valter Longo
Valter Longo, a biogerontologist at USC, and centenarian Rocco Longo (no relation) appear together in Italy in 2021. The elder Longo is from a part of Italy where people have fasted regularly and are enjoying long lifespans.
You’ve probably heard about intermittent fasting, where you don’t eat for about 16 hours each day and limit the window where you’re taking in food to the remaining eight hours.
But there’s another type of fasting, called a fasting-mimicking diet, with studies pointing to important benefits. For today’s podcast episode, I chatted with Dr. Valter Longo, a biogerontologist at the University of Southern California, about all kinds of fasting, and particularly the fasting-mimicking diet, which minimizes hunger as much as possible. Going without food for a period of time is an example of good stress: challenges that work at the cellular level to boost health and longevity.
Listen on Apple | Listen on Spotify | Listen on Stitcher | Listen on Amazon | Listen on Google
If you’ve ever spent more than a few minutes looking into fasting, you’ve almost certainly come upon Dr. Longo's name. He is the author of the bestselling book, The Longevity Diet, and the best known researcher of fasting-mimicking diets.
With intermittent fasting, your body might begin to switch up its fuel type. It's usually running on carbs you get from food, which gets turned into glucose, but without food, your liver starts making something called ketones, which are molecules that may benefit the body in a number of ways.
With the fasting-mimicking diet, you go for several days eating only types of food that, in a way, keep themselves secret from your body. So at the level of your cells, the body still thinks that it’s fasting. This is the best of both worlds – you’re not completely starving because you do take in some food, and you’re getting the boosts to health that come with letting a fast run longer than intermittent fasting. In this episode, Dr. Longo talks about the growing number of studies showing why this could be very advantageous for health, as long as you undertake the diet no more than a few times per year.
Dr. Longo is the director of the Longevity Institute at USC’s Leonard Davis School of Gerontology, and the director of the Longevity and Cancer program at the IFOM Institute of Molecular Oncology in Milan. In addition, he's the founder and president of the Create Cures Foundation in L.A., which focuses on nutrition for the prevention and treatment of major chronic illnesses. In 2016, he received the Glenn Award for Research on Aging for the discovery of genes and dietary interventions that regulate aging and prevent diseases. Dr. Longo received his PhD in biochemistry from UCLA and completed his postdoc in the neurobiology of aging and Alzheimer’s at USC.
Show links:
Create Cures Foundation, founded by Dr. Longo: www.createcures.org
Dr. Longo's Facebook: https://www.facebook.com/profvalterlongo/
Dr. Longo's Instagram: https://www.instagram.com/prof_valterlongo/
Dr. Longo's book: The Longevity Diet
The USC Longevity Institute: https://gero.usc.edu/longevity-institute/
Dr. Longo's research on nutrition, longevity and disease: https://pubmed.ncbi.nlm.nih.gov/35487190/
Dr. Longo's research on fasting mimicking diet and cancer: https://pubmed.ncbi.nlm.nih.gov/34707136/
Full list of Dr. Longo's studies: https://pubmed.ncbi.nlm.nih.gov/?term=Longo%2C+Valter%5BAuthor%5D&sort=date
Research on MCT oil and Alzheimer's: https://alz-journals.onlinelibrary.wiley.com/doi/f...
Keto Mojo device for measuring ketones
Silkworms with spider DNA spin silk stronger than Kevlar
Silkworm silk is fragile, which limits its uses, but a few extra genes can help.
Story by Freethink
The study and copying of nature’s models, systems, or elements to address complex human challenges is known as “biomimetics.” Five hundred years ago, an elderly Italian polymath spent months looking at the soaring flight of birds. The result was Leonardo da Vinci’s biomimetic Codex on the Flight of Birds, one of the foundational texts in the science of aerodynamics. It’s the science that elevated the Wright Brothers and has yet to peak.
Today, biomimetics is everywhere. Shark-inspired swimming trunks, gecko-inspired adhesives, and lotus-inspired water-repellents are all taken from observing the natural world. After millions of years of evolution, nature has quite a few tricks up its sleeve. They are tricks we can learn from. And now, thanks to some spider DNA and clever genetic engineering, we have another one to add to the list.
The elusive spider silk
We’ve known for a long time that spider silk is remarkable, in ways that synthetic fibers can’t emulate. Nylon is incredibly strong (it can support a lot of force), and Kevlar is incredibly tough (it can absorb a lot of force). But neither is both strong and tough. In all artificial polymeric fibers, strength and toughness are mutually exclusive, and so we pick the material best for the job and make do.
Spider silk, a natural polymeric fiber, breaks this rule. It is somehow both strong and tough. No surprise, then, that spider silk is a source of much study.The problem, though, is that spiders are incredibly hard to cultivate — let alone farm. If you put them together, they will attack and kill each other until only one or a few survive. If you put 100 spiders in an enclosed space, they will go about an aggressive, arachnocidal Hunger Games. You need to give each its own space and boundaries, and a spider hotel is hard and costly. Silkworms, on the other hand, are peaceful and productive. They’ll hang around all day to make the silk that has been used in textiles for centuries. But silkworm silk is fragile. It has very limited use.
The elusive – and lucrative – trick, then, would be to genetically engineer a silkworm to produce spider-quality silk. So far, efforts have been fruitless. That is, until now.
We can have silkworms creating silk six times as tough as Kevlar and ten times as strong as nylon.
Spider-silkworms
Junpeng Mi and his colleagues working at Donghua University, China, used CRISPR gene-editing technology to recode the silk-creating properties of a silkworm. First, they took genes from Araneus ventricosus, an East Asian orb-weaving spider known for its strong silk. Then they placed these complex genes – genes that involve more than 100 amino acids – into silkworm egg cells. (This description fails to capture how time-consuming, technical, and laborious this was; it’s a procedure that requires hundreds of thousands of microinjections.)
This had all been done before, and this had failed before. Where Mi and his team succeeded was using a concept called “localization.” Localization involves narrowing in on a very specific location in a genome. For this experiment, the team from Donghua University developed a “minimal basic structure model” of silkworm silk, which guided the genetic modifications. They wanted to make sure they had the exactly right transgenic spider silk proteins. Mi said that combining localization with this basic structure model “represents a significant departure from previous research.” And, judging only from the results, he might be right. Their “fibers exhibited impressive tensile strength (1,299 MPa) and toughness (319 MJ/m3), surpassing Kevlar’s toughness 6-fold.”
A world of super-materials
Mi’s research represents the bursting of a barrier. It opens up hugely important avenues for future biomimetic materials. As Mi puts it, “This groundbreaking achievement effectively resolves the scientific, technical, and engineering challenges that have hindered the commercialization of spider silk, positioning it as a viable alternative to commercially synthesized fibers like nylon and contributing to the advancement of ecological civilization.”
Around 60 percent of our clothing is made from synthetic fibers like nylon, polyester, and acrylic. These plastics are useful, but often bad for the environment. They shed into our waterways and sometimes damage wildlife. The production of these fibers is a source of greenhouse gas emissions. Now, we have a “sustainable, eco-friendly high-strength and ultra-tough alternative.” We can have silkworms creating silk six times as tough as Kevlar and ten times as strong as nylon.
We shouldn’t get carried away. This isn’t going to transform the textiles industry overnight. Gene-edited silkworms are still only going to produce a comparatively small amount of silk – even if farmed in the millions. But, as Mi himself concedes, this is only the beginning. If Mi’s localization and structure-model techniques are as remarkable as they seem, then this opens up the door to a great many supermaterials.
Nature continues to inspire. We had the bird, the gecko, and the shark. Now we have the spider-silkworm. What new secrets will we unravel in the future? And in what exciting ways will it change the world?
This article originally appeared on Freethink, home of the brightest minds and biggest ideas of all time.