Will Blockchain Technology Usher in a Healthcare Data Revolution?
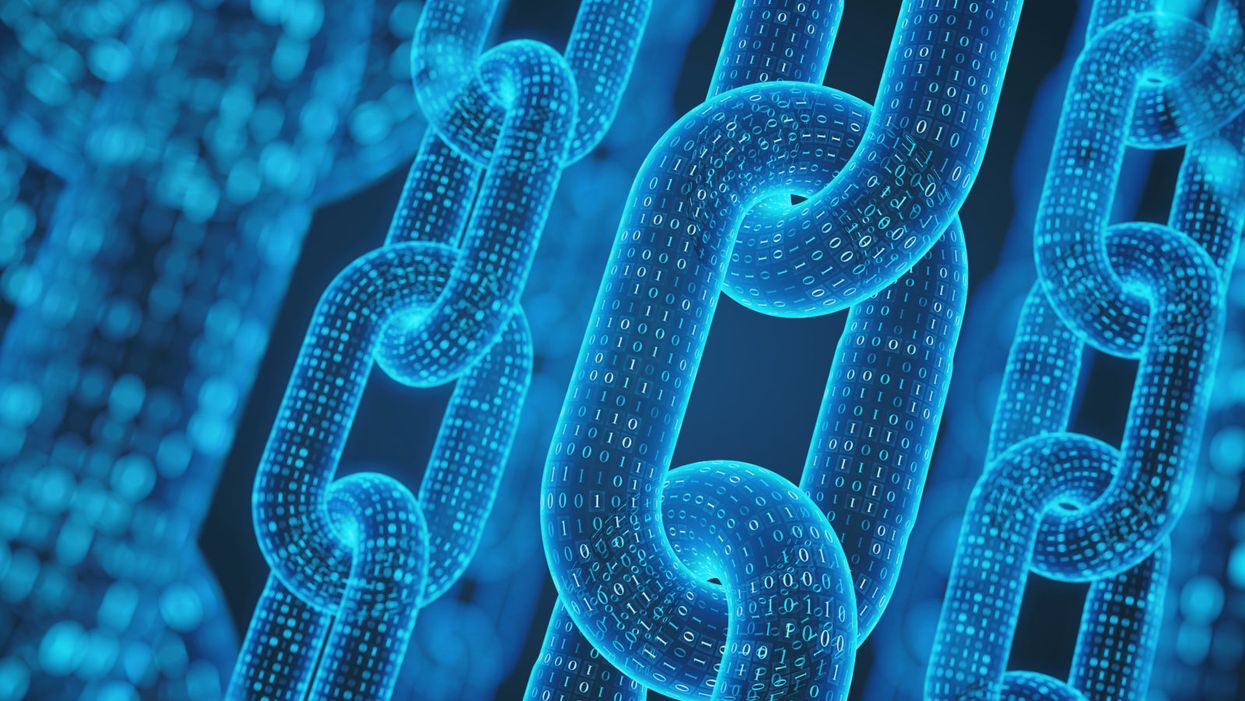
Digital blockchain concept.
The hacker collective known as the Dark Overlord first surfaced in June 2016, when it advertised more than 600,000 patient files from three U.S. healthcare organizations for sale on the dark web. The group, which also attempted to extort ransom from its victims, soon offered another 9 million records pilfered from health insurance companies and provider networks across the country.
Since 2009, federal regulators have counted nearly 5,000 major data breaches in the United States alone, affecting some 260 million individuals.
Last October, apparently seeking publicity as well as cash, the hackers stole a trove of potentially scandalous data from a celebrity plastic surgery clinic in London—including photos of in-progress genitalia- and breast-enhancement surgeries. "We have TBs [terabytes] of this shit. Databases, names, everything," a gang representative told a reporter. "There are some royal families in here."
Bandits like these are prowling healthcare's digital highways in growing numbers. Since 2009, federal regulators have counted nearly 5,000 major data breaches in the United States alone, affecting some 260 million individuals. Although hacker incidents represent less than 20 percent of the total breaches, they account for almost 80 percent of the affected patients. Such attacks expose patients to potential blackmail or identity theft, enable criminals to commit medical fraud or file false tax returns, and may even allow hostile state actors to sabotage electric grids or other infrastructure by e-mailing employees malware disguised as medical notices. According to the consulting agency Accenture, data theft will cost the healthcare industry $305 billion between 2015 and 2019, with annual totals doubling from $40 billion to $80 billion.
Blockchain could put patients in control of their own data, empowering them to access, share, and even sell their medical information as they see fit.
One possible solution to this crisis involves radically retooling the way healthcare data is stored and shared—by using blockchain, the still-emerging information technology that underlies cryptocurrencies such as Bitcoin. And blockchain-enabled IT systems, boosters say, could do much more than prevent the theft of medical data. Such networks could revolutionize healthcare delivery on many levels, creating efficiencies that would reduce medical errors, improve coordination between providers, drive down costs, and give researchers unprecedented insights into patterns of disease. Perhaps most transformative, blockchain could put patients in control of their own data, empowering them to access, share, and even sell their medical information as they see fit. Widespread adoption could result in "a new kind of healthcare economy, in which data and services are quantifiable and exchangeable, with strong guarantees around both the security and privacy of sensitive information," wrote W. Brian Smith, chief scientist of healthcare-blockchain startup PokitDok, in a recent white paper.
Around the world, entrepreneurs, corporations, and government agencies are hopping aboard the blockchain train. A survey by the IBM Institute for Business Value, released in late 2016, found that 16 percent of healthcare executives in 16 countries planned to begin implementing some form of the technology in the coming year; 90 percent planned to launch a pilot program in the next two years. In 2017, Estonia became the first country to switch its medical-records system to a blockchain-based framework. Great Britain and Dubai are exploring a similar move. Yet in countries with more fragmented health systems, most notably the U.S., the challenges remain formidable. Some of the most advanced healthcare applications envisioned for blockchain, moreover, raise technological and ethical questions whose answers may not arrive anytime soon.
By creating a detailed, comprehensive, and immutable timeline of medical transactions, blockchain-based recordkeeping could help providers gauge a patient's long-term health patterns in a way that's never before been possible.
What Exactly Is Blockchain, Anyway?
To understand the buzz around blockchain, it's necessary to grasp (at least loosely) how the technology works. Ordinary digital recordkeeping systems rely on a central administrator that acts as gatekeeper to a treasury of data; if you can sneak past the guard, you can often gain access to the entire hoard, and your intrusion may go undetected indefinitely. Blockchain, by contrast, employs a network of synchronized, replicated databases. Information is scattered among these nodes, rather than on a single server, and is exchanged through encrypted, peer-to-peer pathways. Each transaction is visible to every computer on the network, and must be approved by a majority in order to be successfully completed. Each batch of transactions, or "block," is date- and time-stamped, marked with the user's identity, and given a cryptographic code, which is posted to every node. These blocks form a "chain," preserved in an electronic ledger, that can be read by all users but can't be edited. Any unauthorized access, or attempt at tampering, can be quickly neutralized by these overlapping safeguards. Even if a hacker managed to break into the system, penetrating deeply would be extraordinarily difficult.
Because blockchain technology shares transaction records throughout a network, it could eliminate communication bottlenecks between different components of the healthcare system (primary care physicians, specialists, nurses, and so on). And because blockchain-based systems are designed to incorporate programs known as "smart contracts," which automate functions previously requiring human intervention, they could reduce dangerous slipups as well as tedious and costly paperwork. For example, when a patient gets a checkup, sees a specialist, and fills a prescription, all these actions could be automatically recorded on his or her electronic health record (EHR), checked for errors, submitted for billing, and entered on insurance claims—which could be adjudicated and reimbursed automatically as well. "Blockchain has the potential to remove a lot of intermediaries from existing workflows, whether digital or nondigital," says Kamaljit Behera, an industry analyst for the consulting firm Frost & Sullivan.
The possible upsides don't end there. By creating a detailed, comprehensive, and immutable timeline of medical transactions, blockchain-based recordkeeping could help providers gauge a patient's long-term health patterns in a way that's never before been possible. In addition to data entered by their caregivers, individuals could use app-based technologies or wearables to transmit other information to their records, such as diet, exercise, and sleep patterns, adding new depth to their medical portraits.
Many experts expect healthcare blockchain to take root more slowly in the U.S. than in nations with government-run national health services.
Smart contracts could also allow patients to specify who has access to their data. "If you get an MRI and want your orthopedist to see it, you can add him to your network instead of carrying a CD into his office," explains Andrew Lippman, associate director of the MIT Media Lab, who helped create a prototype healthcare blockchain system called MedRec that's currently being tested at Beth Israel Deaconess Hospital in Boston. "Or you might make a smart contract to allow your son or daughter to access your healthcare records if something happens to you." Another option: permitting researchers to analyze your data for scientific purposes, whether anonymously or with your name attached.
The Recent History, and Looking Ahead
Over the past two years, a crowd of startups has begun vying for a piece of the emerging healthcare blockchain market. Some, like PokitDok and Atlanta-based Patientory, plan to mint proprietary cryptocurrencies, which investors can buy in lieu of stock, medical providers may earn as a reward for achieving better outcomes, and patients might score for meeting wellness goals or participating in clinical trials. (Patientory's initial coin offering, or ICO, raised more than $7 million in three days.) Several fledgling healthcare-blockchain companies have found powerful corporate partners: Intel for Silicon Valley's PokitDok, Kaiser Permanente for Patientory, Philips for Los Angeles-based Gem Health. At least one established provider network, Change Healthcare, is developing blockchain-based systems of its own. Two months ago, Change launched what it calls the first "enterprise-scale" blockchain network in U.S. healthcare—a system to track insurance claim submissions and remittances.
No one, however, has set a roll-out date for a full-blown, blockchain-based EHR system in this country. "We have yet to see anything move from the pilot phase to some kind of production status," says Debbie Bucci, an IT architect in the federal government's Office of the National Coordinator for Health Information Technology. Indeed, many experts expect healthcare blockchain to take root more slowly here than in nations with government-run national health services. In America, a typical patient may have dealings with a family doctor who keeps everything on paper, an assortment of hospitals that use different EHR systems, and an insurer whose system for processing claims is separate from that of the healthcare providers. To help bridge these gaps, a consortium called the Hyperledger Healthcare Working Group (which includes many of the leading players in the field) is developing standard protocols for blockchain interoperability and other functions. Adding to the complexity is the federal Health Insurance and Portability Act (HIPAA), which governs who can access patient data and under what circumstances. "Healthcare blockchain is in a very nascent stage," says Behera. "Coming up with regulations and other guidelines, and achieving large-scale implementation, will take some time."
The ethical implications of buying and selling personal genomic data in an electronic marketplace are doubtless open to debate.
How long? Behera, like other analysts, estimates that relatively simple applications, such as revenue-cycle management systems, could become commonplace in the next five years. More ambitious efforts might reach fruition in a decade or so. But once the infrastructure for healthcare blockchain is fully established, its uses could go far beyond keeping better EHRs.
A handful of scientists and entrepreneurs are already working to develop one visionary application: managing genomic data. Last month, Harvard University geneticist George Church—one of the most influential figures in his discipline—launched a business called Nebula Genomics. It aims to set up an exchange in which individuals can use "Neptune tokens" to purchase DNA sequencing, which will be stored in the company's blockchain-based system; research groups will be able to pay clients for their data using the same cryptocurrency. Luna DNA, founded by a team of biotech veterans in San Diego, plans a similar service, as does a Moscow-based startup called the Zenome Project.
Hossein Rahnama, CEO of the mobile-tech company Flybits and director of research at the Ryerson Centre for Cloud and Context-Aware Computing in Toronto, envisions a more personalized way of sharing genomic data via blockchain. His firm is working with a U.S. insurance company to develop a service that would allow clients in their 20s and 30s to connect with people in their 70s or 80s with similar genomes. The young clients would learn how the elders' lifestyle choices had influenced their health, so that they could modify their own habits accordingly. "It's intergenerational wisdom-sharing," explains Rahnama, who is 38. "I would actually pay to be a part of that network."
The ethical implications of buying and selling personal genomic data in an electronic marketplace are doubtless open to debate. Such commerce could greatly expand the pool of subjects for research in many areas of medicine, enabling the kinds of breakthroughs that only Big Data can provide. Yet it could also lead millions to surrender the most private information of all—the secrets of their cells—to buyers with less benign intentions. The Dark Overlord, one might argue, could not hope for a more satisfying victory.
These scenarios, however, are pure conjecture. After the first web page was posted, in 1991, Lippman observes, "a whole universe developed that you couldn't have imagined on Day 1." The same, he adds, is likely true for healthcare blockchain. "Our vision is to make medical records useful for you and for society, and to give you more control over your own identity. Time will tell."
Important findings are starting to emerge from research on how genes shape the human response to the Covid virus.
From infections with no symptoms to why men are more likely to be hospitalized in the ICU and die of COVID-19, new research shows that your genes play a significant role
Early in the pandemic, genetic research focused on the virus because it was readily available. Plus, the virus contains only 30,000 bases in a dozen functional genes, so it's relatively easy and affordable to sequence. Additionally, the rapid mutation of the virus and its ability to escape antibody control fueled waves of different variants and provided a reason to follow viral genetics.
In comparison, there are many more genes of the human immune system and cellular functions that affect viral replication, with about 3.2 billion base pairs. Human studies require samples from large numbers of people, the analysis of each sample is vastly more complex, and sophisticated computer analysis often is required to make sense of the raw data. All of this takes time and large amounts of money, but important findings are beginning to emerge.
Asymptomatics
About half the people exposed to SARS-CoV-2, the virus that causes the COVID-19 disease, never develop symptoms of this disease, or their symptoms are so mild they often go unnoticed. One piece of understanding the phenomena came when researchers showed that exposure to OC43, a common coronavirus that results in symptoms of a cold, generates immune system T cells that also help protect against SARS-CoV-2.
Jill Hollenbach, an immunologist at the University of California at San Francisco, sought to identify the gene behind that immune protection. Most COVID-19 genetic studies are done with the most seriously ill patients because they are hospitalized and thus available. “But 99 percent of people who get it will never see the inside of a hospital for COVID-19,” she says. “They are home, they are not interacting with the health care system.”
Early in the pandemic, when most labs were shut down, she tapped into the National Bone Marrow Donor Program database. It contains detailed information on donor human leukocyte antigens (HLAs), key genes in the immune system that must match up between donor and recipient for successful transplants of marrow or organs. Each HLA can contain alleles, slight molecular differences in the DNA of the HLA, which can affect its function. Potential HLA combinations can number in the tens of thousands across the world, says Hollenbach, but each person has a smaller number of those possible variants.
She teamed up with the COVID-19 Citizen Science Study a smartphone-based study to track COVID-19 symptoms and outcomes, to ask persons in the bone marrow donor registry about COVID-19. The study enlisted more than 30,000 volunteers. Those volunteers already had their HLAs annotated by the registry, and 1,428 tested positive for the virus.
Analyzing five key HLAs, she found an allele in the gene HLA-B*15:01 that was significantly overrepresented in people who didn’t have any symptoms. The effect was even stronger if a person had inherited the allele from both parents; these persons were “more than eight times more likely to remain asymptomatic than persons who did not carry the genetic variant,” she says. Altogether this HLA was present in about 10 percent of the general European population but double that percentage in the asymptomatic group. Hollenbach and her colleagues were able confirm this in other different groups of patients.
What made the allele so potent against SARS-CoV-2? Part of the answer came from x-ray crystallography. A key element was the molecular shape of parts of the cold virus OC43 and SARS-CoV-2. They were virtually identical, and the allele could bind very tightly to them, present their molecular antigens to T cells, and generate an extremely potent T cell response to the viruses. And “for whatever reasons that generated a lot of memory T cells that are going to stick around for a long time,” says Hollenbach. “This T cell response is very early in infection and ramps up very quickly, even before the antibody response.”
Understanding the genetics of the immune response to SARS-CoV-2 is important because it provides clues into the conditions of T cells and antigens that support a response without any symptoms, she says. “It gives us an opportunity to think about whether this might be a vaccine design strategy.”
Dead men
A researcher at the Leibniz Institute of Virology in Hamburg Germany, Guelsah Gabriel, was drawn to a question at the other end of the COVID-19 spectrum: why men more likely to be hospitalized and die from the infection. It wasn't that men were any more likely to be exposed to the virus but more likely, how their immune system reacted to it
Several studies had noted that testosterone levels were significantly lower in men hospitalized with COVID-19. And, in general, the lower the testosterone, the worse the prognosis. A year after recovery, about 30 percent of men still had lower than normal levels of testosterone, a condition known as hypogonadism. Most of the men also had elevated levels of estradiol, a female hormone (https://pubmed.ncbi.nlm.nih.gov/34402750/).
Every cell has a sex, expressing receptors for male and female hormones on their surface. Hormones docking with these receptors affect the cells' internal function and the signals they send to other cells. The number and role of these receptors varies from tissue to tissue.
Gabriel began her search by examining whole exome sequences, the protein-coding part of the genome, for key enzymes involved in the metabolism of sex hormones. The research team quickly zeroed in on CYP19A1, an enzyme that converts testosterone to estradiol. The gene that produces this enzyme has a number of different alleles, the molecular variants that affect the enzyme's rate of metabolizing the sex hormones. One genetic variant, CYP19A1 (Thr201Met), is typically found in 6.2 percent of all people, both men and women, but remarkably, they found it in 68.7 percent of men who were hospitalized with COVID-19.
Lung surprise
Lungs are the tissue most affected in COVID-19 disease. Gabriel wondered if the virus might be affecting expression of their target gene in the lung so that it produces more of the enzyme that converts testosterone to estradiol. Studying cells in a petri dish, they saw no change in gene expression when they infected cells of lung tissue with influenza and the original SARS-CoV viruses that caused the SARS outbreak in 2002. But exposure to SARS-CoV-2, the virus responsible for COVID-19, increased gene expression up to 40-fold, Gabriel says.
Did the same thing happen in humans? Autopsy examination of patients in three different cites found that “CYP19A1 was abundantly expressed in the lungs of COVID-19 males but not those who died of other respiratory infections,” says Gabriel. This increased enzyme production led likely to higher levels of estradiol in the lungs of men, which “is highly inflammatory, damages the tissue, and can result in fibrosis or scarring that inhibits lung function and repair long after the virus itself has disappeared.” Somehow the virus had acquired the capacity to upregulate expression of CYP19A1.
Only two COVID-19 positive females showed increased expression of this gene. The menopause status of these women, or whether they were on hormone replacement therapy was not known. That could be important because female hormones have a protective effect for cardiovascular disease, which women often lose after going through menopause, especially if they don’t start hormone replacement therapy. That sex-specific protection might also extend to COVID-19 and merits further study.
The team was able to confirm their findings in golden hamsters, the animal model of choice for studying COVID-19. Testosterone levels in male animals dropped 5-fold three days after infection and began to recover as viral levels declined. CYP19A1 transcription increased up to 15-fold in the lungs of the male but not the females. The study authors wrote, “Virus replication in the male lungs was negatively associated with testosterone levels.”
The medical community studying COVID-19 has slowly come to recognize the importance of adipose tissue, or fat cells. They are known to express abundant levels of CYP19A1 and play a significant role as metabolic tissue in COVID-19. Gabriel adds, “One of the key findings of our study is that upon SARS-CoV-2 infection, the lung suddenly turns into a metabolic organ by highly expressing” CYP19A1.
She also found evidence that SARS-CoV-2 can infect the gonads of hamsters, thereby likely depressing circulating levels of sex hormones. The researchers did not have autopsy samples to confirm this in humans, but others have shown that the virus can replicate in those tissues.
A possible treatment
Back in the lab, substituting low and high doses of testosterone in SARS-COV-2 infected male hamsters had opposite effects depending on testosterone dosage used. Gabriel says that hormone levels can vary so much, depending on health status and age and even may change throughout the day, that “it probably is much better to inhibit the enzyme” produced by CYP19A1 than try to balance the hormones.
Results were better with letrozole, a drug approved to treat hypogonadism in males, which reduces estradiol levels. The drug also showed benefit in male hamsters in terms of less severe disease and faster recovery. She says more details need to be worked out in using letrozole to treat COVID-19, but they are talking with hospitals about clinical trials of the drug.
Gabriel has proposed a four hit explanation of how COVID-19 can be so deadly for men: the metabolic quartet. First is the genetic risk factor of CYP19A1 (Thr201Met), then comes SARS-CoV-2 infection that induces even greater expression of this gene and the deleterious increase of estradiol in the lung. Age-related hypogonadism and the heightened inflammation of obesity, known to affect CYP19A1 activity, are contributing factors in this deadly perfect storm of events.
Studying host genetics, says Gabriel, can reveal new mechanisms that yield promising avenues for further study. It’s also uniting different fields of science into a new, collaborative approach they’re calling “infection endocrinology,” she says.
New device finds breast cancer like earthquake detection
Jessica Fitzjohn, a postdoctoral fellow at the University of Canterbury, demonstrates the novel breast cancer screening device.
Mammograms are necessary breast cancer checks for women as they reach the recommended screening age between 40 and 50 years. Yet, many find the procedure uncomfortable. “I have large breasts, and to be able to image the full breast, the radiographer had to manipulate my breast within the machine, which took time and was quite uncomfortable,” recalls Angela, who preferred not to disclose her last name.
Breast cancer is the most widespread cancer in the world, affecting 2.3 million women in 2020. Screening exams such as mammograms can help find breast cancer early, leading to timely diagnosis and treatment. If this type of cancer is detected before the disease has spread, the 5-year survival rate is 99 percent. But some women forgo mammograms due to concerns about radiation or painful compression of breasts. Other issues, such as low income and a lack of access to healthcare, can also serve as barriers, especially for underserved populations.
Researchers at the University of Canterbury and startup Tiro Medical in Christchurch, New Zealand are hoping their new device—which doesn’t involve any radiation or compression of the breasts—could increase the accuracy of breast cancer screening, broaden access and encourage more women to get checked. They’re digging into clues from the way buildings move in an earthquake to help detect more cases of this disease.
Earthquake engineering inspires new breast cancer screening tech
What’s underneath a surface affects how it vibrates. Earthquake engineers look at the vibrations of swaying buildings to identify the underlying soil and tissue properties. “As the vibration wave travels, it reflects the stiffness of the material between that wave and the surface,” says Geoff Chase, professor of engineering at the University of Canterbury in Christchurch, New Zealand.
Chase is applying this same concept to breasts. Analyzing the surface motion of the breast as it vibrates could reveal the stiffness of the tissues underneath. Regions of high stiffness could point to cancer, given that cancerous breast tissue can be up to 20 times stiffer than normal tissue. “If in essence every woman’s breast is soft soil, then if you have some granite rocks in there, we’re going to see that on the surface,” explains Chase.
The earthquake-inspired device exceeds the 87 percent sensitivity of a 3D mammogram.
That notion underpins a new breast screening device, the brainchild of Chase. Women lie face down, with their breast being screened inside a circular hole and the nipple resting on a small disc called an actuator. The actuator moves up and down, between one and two millimeters, so there’s a small vibration, “almost like having your phone vibrate on your nipple,” says Jessica Fitzjohn, a postdoctoral fellow at the University of Canterbury who collaborated on the device design with Chase.
Cameras surrounding the device take photos of the breast surface motion as it vibrates. The photos are fed into image processing algorithms that convert them into data points. Then, diagnostic algorithms analyze those data points to find any differences in the breast tissue. “We’re looking for that stiffness contrast which could indicate a tumor,” Fitzjohn says.
A nascent yet promising technology
The device has been tested in a clinical trial of 14 women: one with healthy breasts and 13 with a tumor in one breast. The cohort was small but diverse, varying in age, breast volume and tumor size.
Results from the trial yielded a sensitivity rate, or the likelihood of correctly detecting breast cancer, of 85 percent. Meanwhile, the device’s specificity rate, or the probability of diagnosing healthy breasts, was 77 percent. By combining and optimizing certain diagnostic algorithms, the device reached between 92 and 100 percent sensitivity and between 80 and 86 percent specificity, which is comparable to the latest 3D mammogram technology. Called tomosynthesis, these 3D mammograms take a number of sharper, clearer and more detailed 3D images compared to the single 2D image of a conventional mammogram, and have a specificity score of 92 percent. Although the earthquake-inspired device’s specificity is lower, it exceeds the 87 percent sensitivity of a 3D mammogram.
The team hopes that cameras with better resolution can help improve the numbers. And with a limited amount of data in the first trial, the researchers are looking into funding for another clinical trial to validate their results on a larger cohort size.
Additionally, during the trial, the device correctly identified one woman’s breast as healthy, while her prior mammogram gave a false positive. The device correctly identified it as being healthy tissue. It was also able to capture the tiniest tumor at 7 millimeters—around a third of an inch or half as long as an aspirin tablet.
Diagnostic findings from the device are immediate.
When using the earthquake-inspired device, women lie face down, with their breast being screened inside circular holes.
University of Canterbury.
But more testing is needed to “prove the device’s ability to pick up small breast cancers less than 10 to 15 millimeters in size, as we know that finding cancers when they are small is the best way of improving outcomes,” says Richard Annand, a radiologist at Pacific Radiology in New Zealand. He explains that mammography already detects most precancerous lesions, so if the device will only be able to find large masses or lumps it won’t be particularly useful. While not directly involved in administering the clinical trial for the device, Annand was a director at the time for Canterbury Breastcare, where the trial occurred.
Meanwhile, Monique Gary, a breast surgical oncologist and medical director of the Grand View Health Cancer program in Pennsylvania, U.S., is excited to see new technologies advancing breast cancer screening and early detection. But she notes that the device may be challenging for “patients who are unable to lay prone, such as pregnant women as well as those who are differently abled, and this machine might exclude them.” She adds that it would also be interesting to explore how breast implants would impact the device’s vibrational frequency.
Diagnostic findings from the device are immediate, with the results available “before you put your clothes back on,” Chase says. The absence of any radiation is another benefit, though Annand considers it a minor edge “as we know the radiation dose used in mammography is minimal, and the advantages of having a mammogram far outweigh the potential risk of radiation.”
The researchers also conducted a separate ergonomic trial with 40 women to assess the device’s comfort, safety and ease of use. Angela was part of that trial and described the experience as “easy, quick, painless and required no manual intervention from an operator.” And if a person is uncomfortable being topless or having their breasts touched by someone else, “this type of device would make them more comfortable and less exposed,” she says.
While mammograms remain “the ‘gold standard’ in breast imaging, particularly screening, physicians need an option that can be used in combination with mammography.
Fitzjohn acknowledges that “at the moment, it’s quite a crude prototype—it’s just a block that you lie on.” The team prioritized function over form initially, but they’re now planning a few design improvements, including more cushioning for the breasts and the surface where the women lie on.
While mammograms remains “the ‘gold standard’ in breast imaging, particularly screening, physicians need an option that is good at excluding breast cancer when used in combination with mammography, has good availability, is easy to use and is affordable. There is the possibility that the device could fill this role,” Annand says.
Indeed, the researchers envision their new breast screening device as complementary to mammograms—a prescreening tool that could make breast cancer checks widely available. As the device is portable and doesn’t require specialized knowledge to operate, it can be used in clinics, pop-up screening facilities and rural communities. “If it was easily accessible, particularly as part of a checkup with a [general practitioner] or done in a practice the patient is familiar with, it may encourage more women to access this service,” Angela says. For those who find regular mammograms uncomfortable or can’t afford them, the earthquake-inspired device may be an option—and an even better one.
Broadening access could prompt more women to go for screenings, particularly younger women at higher risk of getting breast cancer because of a family history of the disease or specific gene mutations. “If we can provide an option for them then we can catch those cancers earlier,” Fitzjohn syas. “By taking screening to people, we’re increasing patient-centric care.”
With the team aiming to lower the device’s cost to somewhere between five and eight times less than mammography equipment, it would also be valuable for low-to-middle-income nations that are challenged to afford the infrastructure for mammograms or may not have enough skilled radiologists.
For Fitzjohn, the ultimate goal is to “increase equity in breast screening and catch cancer early so we have better outcomes for women who are diagnosed with breast cancer.”