With Lab-Grown Chicken Nuggets, Dumplings, and Burgers, Futuristic Foods Aim to Seem Familiar
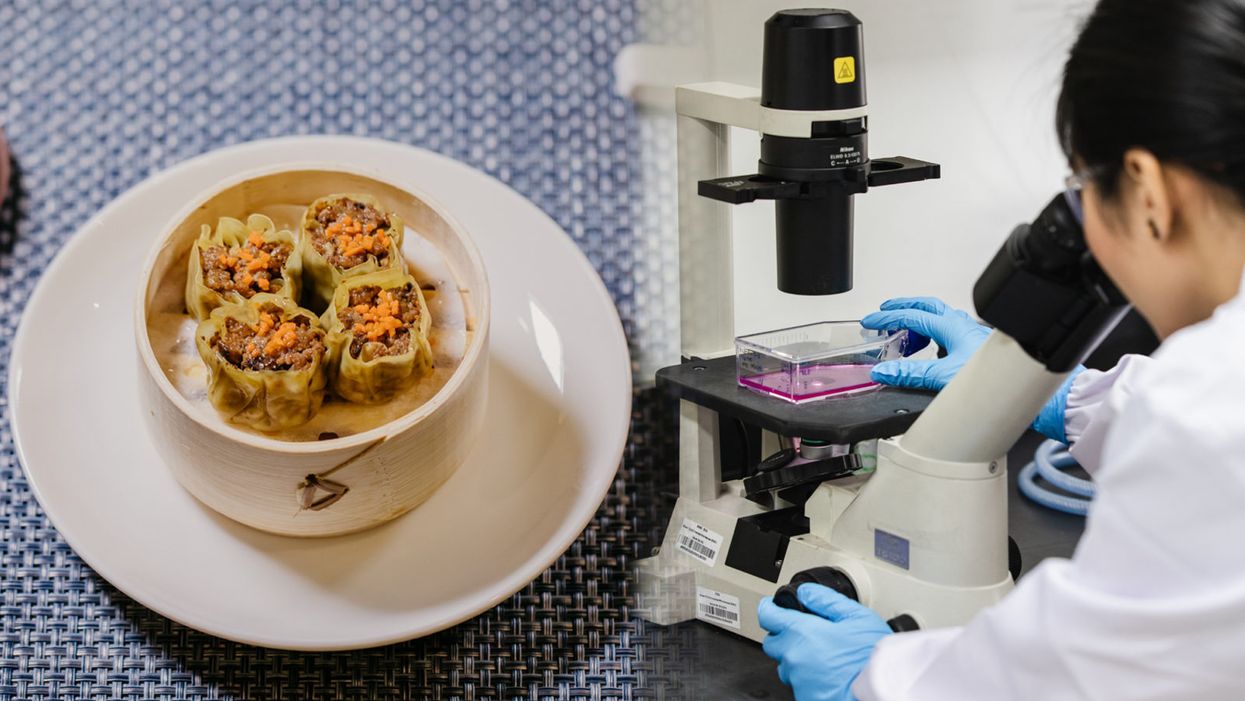
Shiok Meat's lab-grown "shrimp" dumplings, alongside a photo of the company's CTO, Ka Yi Ling, in a lab.
Sandhya Sriram is at the forefront of the expanding lab-grown meat industry in more ways than one.
"[Lab-grown meat] is kind of a brave new world for a lot of people, and food isn't something people like being brave about."
She's the CEO and co-founder of one of fewer than 30 companies that is even in this game in the first place. Her Singapore-based company, Shiok Meats, is the only one to pop up in Southeast Asia. And it's the only company in the world that's attempting to grow crustaceans in a lab, starting with shrimp. This spring, the company debuted a prototype of its shrimp, and completed a seed funding round of $4.6 million.
Yet despite all of these wins, Sriram's own mother won't try the company's shrimp. She's a staunch, lifelong vegetarian, adhering to a strict definition of what that means.
"[Lab-grown meat] is kind of a brave new world for a lot of people, and food isn't something people like being brave about. It's really a rather hard-wired thing," says Kate Krueger, the research director at New Harvest, a non-profit accelerator for cellular agriculture (the umbrella field that studies how to grow animal products in the lab, including meat, dairy, and eggs).
It's so hard-wired, in fact, that trends in food inform our species' origin story. In 2017, a group of paleoanthropologists caused an upset when they unearthed fossils in present day Morocco showing that our earliest human ancestors lived much further north and 100,000 years earlier than expected -- the remains date back 300,000 years. But the excavation not only included bones and tools, it also painted a clear picture of the prevailing menu at the time: The oldest humans were apparently chomping on tons of gazelle, as well as wildebeest and zebra when they could find them, plus the occasional seasonal ostrich egg.
These were people with a diet shaped by available resources, but also by the ability to cook in the first place. In his book Catching Fire: How Cooking Made Us Human, Harvard primatologist Richard Wrangam writes that the very thing that allowed for the evolution of Homo sapiens was the ability to transform raw ingredients into edible nutrients through cooking.
Today, our behavior and feelings around food are the product of local climate, crops, animal populations, and tools, but also religion, tradition, and superstition. So what happens when you add science to the mix? Turns out, we still trend toward the familiar. The innovations in lab-grown meat that are picking up the most steam are foods like burgers, not meat chips, and salmon, not salmon-cod-tilapia hybrids. It's not for lack of imagination, it's because the industry's practitioners know that a lifetime of food memories is a hard thing to contend with. So far, the nascent lab-grown meat industry is not so much disrupting as being shaped by the oldest culture we have.
Not a single piece of lab-grown meat is commercially available to consumers yet, and already so much ink has been spilled debating if it's really meat, if it's kosher, if it's vegetarian, if it's ethical, if it's sustainable. But whether or not the industry succeeds and sticks around is almost moot -- watching these conversations and innovations unfold serves as a mirror reflecting back who we are, what concerns us, and what we aspire to.
The More Things Change, the More They Stay the Same
The building blocks for making lab-grown meat right now are remarkably similar, no matter what type of animal protein a company is aiming to produce.
First, a small biopsy, about the size of a sesame seed, is taken from a single animal. Then, the muscle cells are isolated and added to a nutrient-dense culture in a bioreactor -- the same tool used to make beer -- where the cells can multiply, grow, and form muscle tissue. This tissue can then be mixed with additives like nutrients, seasonings, binders, and sometimes colors to form a food product. Whether a company is attempting to make chicken, fish, beef, shrimp, or any other animal protein in a lab, the basic steps remain similar. Cells from various animals do behave differently, though, and each company has its own proprietary techniques and tools. Some, for example, use fetal calf serum as their cell culture, while others, aiming for a more vegan approach, eschew it.
"New gadgets feel safest when they remind us of other objects that we already know."
According to Mark Post, who made the first lab-grown hamburger at Maastricht University in the Netherlands in 2013, the cells of just one cow can give way to 175 million four-ounce burgers. By today's available burger-making methods, you'd need to slaughter 440,000 cows for the same result. The projected difference in the purely material efficiency between the two systems is staggering. The environmental impact is hard to predict, though. Some companies claim that their lab-grown meat requires 99 percent less land and 96 percent less water than traditional farming methods -- and that rearing fewer cows, specifically, would reduce methane emissions -- but the energy cost of running a lab-grown-meat production facility at an industrial scale, especially as compared to small-scale, pasture-raised farming, could be problematic. It's difficult to truly measure any of this in a burgeoning industry.
At this point, growing something like an intact shrimp tail or a marbled steak in a lab is still a Holy Grail. It would require reproducing the complex musculo-skeletal and vascular structure of meat, not just the cellular basis, and no one's successfully done it yet. Until then, many companies working on lab-grown meat are perfecting mince. Each new company's demo of a prototype food feels distinctly regional, though: At the Disruption in Food and Sustainability Summit in March, Shiok (which is pronounced "shook," and is Singaporean slang for "very tasty and delicious") first shared a prototype of its shrimp as an ingredient in siu-mai, a dumpling of Chinese origin and a fixture at dim sum. JUST, a company based in the U.S., produced a demo chicken nugget.
As Jean Anthelme Brillat-Savarin, the 17th century founder of the gastronomic essay, famously said, "Show me what you eat, and I'll tell you who you are."
For many of these companies, the baseline animal protein they are trying to innovate also feels tied to place and culture: When meat comes from a bioreactor, not a farm, the world's largest exporter of seafood could be a landlocked region, and beef could be "reared" in a bayou, yet the handful of lab-grown fish companies, like Finless Foods and BlueNalu, hug the American coasts; VOW, based in Australia, started making lab-grown kangaroo meat in August; and of course the world's first lab-grown shrimp is in Singapore.
"In the U.S., shrimps are either seen in shrimp cocktail, shrimp sushi, and so on, but [in Singapore] we have everything from shrimp paste to shrimp oil," Sriram says. "It's used in noodles and rice, as flavoring in cup noodles, and in biscuits and crackers as well. It's seen in every form, shape, and size. It just made sense for us to go after a protein that was widely used."
It's tempting to assume that innovating on pillars of cultural significance might be easier if the focus were on a whole new kind of food to begin with, not your popular dim sum items or fast food offerings. But it's proving to be quite the opposite.
"That could have been one direction where [researchers] just said, 'Look, it's really hard to reproduce raw ground beef. Why don't we just make something completely new, like meat chips?'" says Mike Lee, co-founder and co-CEO of Alpha Food Labs, which works on food innovation more broadly. "While that strategy's interesting, I think we've got so many new things to explain to people that I don't know if you want to also explain this new format of food that you've never, ever seen before."
We've seen this same cautious approach to change before in other ways that relate to cooking. Perhaps the most obvious example is the kitchen range. As Bee Wilson writes in her book Consider the Fork: A History of How We Cook and Eat, in the 1880s, convincing ardent coal-range users to switch to newfangled gas was a hard sell. To win them over, inventor William Sugg designed a range that used gas, but aesthetically looked like the coal ones already in fashion at the time -- and which in some visual ways harkened even further back to the days of open-hearth cooking. Over time, gas range designs moved further away from those of the past, but the initial jump was only made possible through familiarity. There's a cleverness to meeting people where they are.
"New gadgets feel safest when they remind us of other objects that we already know," writes Wilson. "It is far harder to accept a technology that is entirely new."
Maybe someday we won't want anything other than meat chips, but not today.
Measuring Success
A 2018 Gallup poll shows that in the U.S., rates of true vegetarianism and veganism have been stagnant for as long as they've been measured. When the poll began in 1999, six percent of Americans were vegetarian, a number that remained steady until 2012, when the number dropped one point. As of 2018, it remained at five percent.
In 2012, when Gallup first measured the percentage of vegans, the rate was two percent. By 2018 it had gone up just one point, to three percent. Increasing awareness of animal welfare, health, and environmental concerns don't seem to be incentive enough to convince Americans, en masse, to completely slam the door on a food culture characterized in many ways by its emphasis on traditional meat consumption.
"A lot of consumers get over the ick factor when you tell them that most of the food that you're eating right now has entered the lab at some point."
Wilson writes that "experimenting with new foods has always been a dangerous business. In the wild, trying out some tempting new berries might lead to death. A lingering sense of this danger may make us risk-averse in the kitchen."
That might be one psychologically deep-seated reason that Americans are so resistant to ditch meat altogether. But a middle ground is emerging with a rise in flexitarianism, which aims to reduce reliance on traditional animal products. "Americans are eager to include alternatives to animal products in their diets, but are not willing to give up animal products completely," the same 2018 Gallup poll reported. This may represent the best opportunity for lab-grown meat to wedge itself into the culture.
Quantitatively predicting a population's willingness to try a lab-grown version of its favorite protein is proving a hard thing to measure, however, because it's still science fiction to a regular consumer. Measuring popular opinion of something that doesn't really exist yet is a dubious pastime.
In 2015, University of Wisconsin School of Public Health researchers Linnea Laestadius and Mark Caldwell conducted a study using online comments on articles about lab-grown meat to suss out public response to the food. The results showed a mostly negative attitude, but that was only two years into a field that is six years old today. Already public opinion may have shifted.
Shiok Meat's Sriram and her co-founder Ka Yi Ling have used online surveys to get a sense of the landscape, but they also take a more direct approach sometimes. Every time they give a public talk about their company and their shrimp, they poll their audience before and after the talk, using the question, "How many of you are willing to try, and pay, to eat lab-grown meat?"
They consistently find that the percentage of people willing to try goes up from 50 to 90 percent after hearing their talk, which includes information about the downsides of traditional shrimp farming (for one thing, many shrimp are raised in sewage, and peeled and deveined by slaves) and a bit of information about how lab-grown animal protein is being made now. I saw this pan out myself when Ling spoke at a New Harvest conference in Cambridge, Massachusetts in July.
"A lot of consumers get over the ick factor when you tell them that most of the food that you're eating right now has entered the lab at some point," Sriram says. "We're not going to grow our meat in the lab always. It's in the lab right now, because we're in R&D. Once we go into manufacturing ... it's going to be a food manufacturing facility, where a lot of food comes from."
The downside of the University of Wisconsin's and Shiok Meat's approach to capturing public opinion is that they each look at self-selecting groups: Online commenters are often fueled by a need to complain, and it's likely that anyone attending a talk by the co-founders of a lab-grown meat company already has some level of open-mindedness.
So Sriram says that she and Ling are also using another method to assess the landscape, and it's somewhere in the middle. They've been watching public responses to the closest available product to lab-grown meat that's on the market: Impossible Burger. As a 100 percent plant-based burger, it's not quite the same, but this bleedable, searable patty is still very much the product of science and laboratory work. Its remarkable similarity to beef is courtesy of yeast that have been genetically engineered to contain DNA from soy plant roots, which produce a protein called heme as they multiply. This heme is a plant-derived protein that can look and act like the heme found in animal muscle.
So far, the sciencey underpinnings of the burger don't seem to be turning people off. In just four years, it's already found its place within other American food icons. It's readily available everywhere from nationwide Burger Kings to Boston's Warren Tavern, which has been in operation since 1780, is one of the oldest pubs in America, and is even named after the man who sent Paul Revere on his midnight ride. Some people have already grown so attached to the Impossible Burger that they will actually walk out of a restaurant that's out of stock. Demand for the burger is outpacing production.
"Even though [Impossible] doesn't consider their product cellular agriculture, it's part of a spectrum of innovation," Krueger says. "There are novel proteins that you're not going to find in your average food, and there's some cool tech there. So to me, that does show a lot of willingness on people's part to think about trying something new."
The message for those working on animal-based lab-grown meat is clear: People will accept innovation on their favorite food if it tastes good enough and evokes the same emotional connection as the real deal.
"How people talk about lab-grown meat now, it's still a conversation about science, not about culture and emotion," Lee says. But he's confident that the conversation will start to shift in that direction if the companies doing this work can nail the flavor memory, above all.
And then proving how much power flavor lords over us, we quickly derail into a conversation about Doritos, which he calls "maniacally delicious." The chips carry no health value whatsoever and are a native product of food engineering and manufacturing — just watch how hard it is for Bon Appetit associate food editor Claire Saffitz to try and recreate them in the magazine's test kitchen — yet devotees remain unfazed and crunch on.
"It's funny because it shows you that people don't ask questions about how [some foods] are made, so why are they asking so many questions about how lab-grown meat is made?" Lee asks.
For all the hype around Impossible Burger, there are still controversies and hand-wringing around lab-grown meat. Some people are grossed out by the idea, some people are confused, and if you're the U.S. Cattlemen's Association (USCA), you're territorial. Last year, the group sent a petition to the USDA to "exclude products not derived directly from animals raised and slaughtered from the definition of 'beef' and meat.'"
"I think we are probably three or four big food safety scares away from everyone, especially younger generations, embracing lab-grown meat as like, 'Science is good; nature is dirty, and can kill you.'"
"I have this working hypothesis that if you look at the nation in 50-year spurts, we revolve back and forth between artisanal, all-natural food that's unadulterated and pure, and food that's empowered by science," Lee says. "Maybe we've only had one lap around the track on that, but I think we are probably three or four big food safety scares away from everyone, especially younger generations, embracing lab-grown meat as like, 'Science is good; nature is dirty, and can kill you.'"
Food culture goes beyond just the ingredients we know and love — it's also about how we interact with them, produce them, and expect them to taste and feel when we bite down. We accept a margin of difference among a fast food burger, a backyard burger from the grill, and a gourmet burger. Maybe someday we'll accept the difference between a burger created by killing a cow and a burger created by biopsying one.
Looking to the Future
Every time we engage with food, "we are enacting a ritual that binds us to the place we live and to those in our family, both living and dead," Wilson writes in Consider the Fork. "Such things are not easily shrugged off. Every time a new cooking technology has been introduced, however useful … it has been greeted in some quarters with hostility and protestations that the old ways were better and safer."
This is why it might be hard for a vegetarian mother to try her daughter's lab-grown shrimp, no matter how ethically it was produced or how awe-inspiring the invention is. Yet food cultures can and do change. "They're not these static things," says Benjamin Wurgaft, a historian whose book Meat Planet: Artificial Flesh and the Future of Food comes out this month. "The real tension seems to be between slow change and fast change."
In fact, the very definition of the word "meat" has never exclusively meant what the USCA wants it to mean. Before the 12th century, when it first appeared in Old English as "mete," it wasn't very specific at all and could be used to describe anything from "nourishment," to "food item," to "fodder," to "sustenance." By the 13th century it had been narrowed down to mean "flesh of warm-blooded animals killed and used as food." And yet the British mincemeat pie lives on as a sweet Christmas treat full of -- to the surprise of many non-Brits -- spiced, dried fruit. Since 1901, we've also used this word with ease as a general term for anything that's substantive -- as in, "the meat of the matter." There is room for yet more definitions to pile on.
"The conversation [about lab-ground meat] has changed remarkably in the last six years," Wurgaft says. "It has become a conversation about whether or not specific companies will bring a product to market, and that's a really different conversation than asking, 'Should we produce meat in the lab?'"
As part of the field research for his book, Wurgaft visited the Rijksmuseum Boerhaave, a Dutch museum that specializes in the history of science and medicine. It was 2015, and he was there to see an exhibit on the future of food. Just two years earlier, Mark Post had made that first lab-grown hamburger about a two-and-a-half hour drive south of the museum. When Wurgaft arrived, he found the novel invention, which Post had donated to the museum, already preserved and served up on a dinner plate, the whole outfit protected by plexiglass.
"They put this in the exhibit as if it were already part of the historical records, which to a historian looked really weird," Wurgaft says. "It looked like somebody taking the most recent supercomputer and putting it in a museum exhibit saying, 'This is the supercomputer that changed everything,' as if you were already 100 years in the future, looking back."
It seemed to symbolize an effort to codify a lab-grown hamburger as a matter of Dutch pride, perhaps someday occupying a place in people's hearts right next to the stroopwafel.
"Who's to say that we couldn't get a whole school of how to cook with lab-grown meat?"
Lee likes to imagine that part of the legacy of lab-grown meat, if it succeeds, will be to inspire entirely new fads in cooking -- a step beyond ones like the crab-filled avocado of the 1960s or the pesto of the 1980s in the U.S.
"[Lab-grown meat] is inherently going to be a different quality than anything we've done with an animal," he says. "Look at every cut [of meat] on the sphere today -- each requires a slightly different cooking method to optimize the flavor of that cut. Who's to say that we couldn't get a whole school of how to cook with lab-grown meat?"
At this point, most of us have no way of trying lab-grown meat. It remains exclusively available through sometimes gimmicky demos reserved for investors and the media. But Wurgaft says the stories we tell about this innovation, the articles we write, the films we make, and yes, even the museum exhibits we curate, all hold as much cultural significance as the product itself might someday.
Regenerative medicine has come a long way, baby
After a cloned baby sheep, what started as one of the most controversial areas in medicine is now promising to transform it.
The field of regenerative medicine had a shaky start. In 2002, when news spread about the first cloned animal, Dolly the sheep, a raucous debate ensued. Scary headlines and organized opposition groups put pressure on government leaders, who responded by tightening restrictions on this type of research.
Fast forward to today, and regenerative medicine, which focuses on making unhealthy tissues and organs healthy again, is rewriting the code to healing many disorders, though it’s still young enough to be considered nascent. What started as one of the most controversial areas in medicine is now promising to transform it.
Progress in the lab has addressed previous concerns. Back in the early 2000s, some of the most fervent controversy centered around somatic cell nuclear transfer (SCNT), the process used by scientists to produce Dolly. There was fear that this technique could be used in humans, with possibly adverse effects, considering the many medical problems of the animals who had been cloned.
But today, scientists have discovered better approaches with fewer risks. Pioneers in the field are embracing new possibilities for cellular reprogramming, 3D organ printing, AI collaboration, and even growing organs in space. It could bring a new era of personalized medicine for longer, healthier lives - while potentially sparking new controversies.
Engineering tissues from amniotic fluids
Work in regenerative medicine seeks to reverse damage to organs and tissues by culling, modifying and replacing cells in the human body. Scientists in this field reach deep into the mechanisms of diseases and the breakdowns of cells, the little workhorses that perform all life-giving processes. If cells can’t do their jobs, they take whole organs and systems down with them. Regenerative medicine seeks to harness the power of healthy cells derived from stem cells to do the work that can literally restore patients to a state of health—by giving them healthy, functioning tissues and organs.
Modern-day regenerative medicine takes its origin from the 1998 isolation of human embryonic stem cells, first achieved by John Gearhart at Johns Hopkins University. Gearhart isolated the pluripotent cells that can differentiate into virtually every kind of cell in the human body. There was a raging controversy about the use of these cells in research because at that time they came exclusively from early-stage embryos or fetal tissue.
Back then, the highly controversial SCNT cells were the only way to produce genetically matched stem cells to treat patients. Since then, the picture has changed radically because other sources of highly versatile stem cells have been developed. Today, scientists can derive stem cells from amniotic fluid or reprogram patients’ skin cells back to an immature state, so they can differentiate into whatever types of cells the patient needs.
In the context of medical history, the field of regenerative medicine is progressing at a dizzying speed. But for those living with aggressive or chronic illnesses, it can seem that the wheels of medical progress grind slowly.
The ethical debate has been dialed back and, in the last few decades, the field has produced important innovations, spurring the development of whole new FDA processes and categories, says Anthony Atala, a bioengineer and director of the Wake Forest Institute for Regenerative Medicine. Atala and a large team of researchers have pioneered many of the first applications of 3D printed tissues and organs using cells developed from patients or those obtained from amniotic fluid or placentas.
His lab, considered to be the largest devoted to translational regenerative medicine, is currently working with 40 different engineered human tissues. Sixteen of them have been transplanted into patients. That includes skin, bladders, urethras, muscles, kidneys and vaginal organs, to name just a few.
These achievements are made possible by converging disciplines and technologies, such as cell therapies, bioengineering, gene editing, nanotechnology and 3D printing, to create living tissues and organs for human transplants. Atala is currently overseeing clinical trials to test the safety of tissues and organs engineered in the Wake Forest lab, a significant step toward FDA approval.
In the context of medical history, the field of regenerative medicine is progressing at a dizzying speed. But for those living with aggressive or chronic illnesses, it can seem that the wheels of medical progress grind slowly.
“It’s never fast enough,” Atala says. “We want to get new treatments into the clinic faster, but the reality is that you have to dot all your i’s and cross all your t’s—and rightly so, for the sake of patient safety. People want predictions, but you can never predict how much work it will take to go from conceptualization to utilization.”
As a surgeon, he also treats patients and is able to follow transplant recipients. “At the end of the day, the goal is to get these technologies into patients, and working with the patients is a very rewarding experience,” he says. Will the 3D printed organs ever outrun the shortage of donated organs? “That’s the hope,” Atala says, “but this technology won’t eliminate the need for them in our lifetime.”
New methods are out of this world
Jeanne Loring, another pioneer in the field and director of the Center for Regenerative Medicine at Scripps Research Institute in San Diego, says that investment in regenerative medicine is not only paying off, but is leading to truly personalized medicine, one of the holy grails of modern science.
This is because a patient’s own skin cells can be reprogrammed to become replacements for various malfunctioning cells causing incurable diseases, such as diabetes, heart disease, macular degeneration and Parkinson’s. If the cells are obtained from a source other than the patient, they can be rejected by the immune system. This means that patients need lifelong immunosuppression, which isn’t ideal. “With Covid,” says Loring, “I became acutely aware of the dangers of immunosuppression.” Using the patient’s own cells eliminates that problem.
Microgravity conditions make it easier for the cells to form three-dimensional structures, which could more easily lead to the growing of whole organs. In fact, Loring's own cells have been sent to the ISS for study.
Loring has a special interest in neurons, or brain cells that can be developed by manipulating cells found in the skin. She is looking to eventually treat Parkinson’s disease using them. The manipulated cells produce dopamine, the critical hormone or neurotransmitter lacking in the brains of patients. A company she founded plans to start a Phase I clinical trial using cell therapies for Parkinson’s soon, she says.
This is the culmination of many years of basic research on her part, some of it on her own cells. In 2007, Loring had her own cells reprogrammed, so there’s a cell line that carries her DNA. “They’re just like embryonic stem cells, but personal,” she said.
Loring has another special interest—sending immature cells into space to be studied at the International Space Station. There, microgravity conditions make it easier for the cells to form three-dimensional structures, which could more easily lead to the growing of whole organs. In fact, her own cells have been sent to the ISS for study. “My colleagues and I have completed four missions at the space station,” she says. “The last cells came down last August. They were my own cells reprogrammed into pluripotent cells in 2009. No one else can say that,” she adds.
Future controversies and tipping points
Although the original SCNT debate has calmed down, more controversies may arise, Loring thinks.
One of them could concern growing synthetic embryos. The embryos are ultimately derived from embryonic stem cells, and it’s not clear to what stage these embryos can or will be grown in an artificial uterus—another recent invention. The science, so far done only in animals, is still new and has not been widely publicized but, eventually, “People will notice the production of synthetic embryos and growing them in an artificial uterus,” Loring says. It’s likely to incite many of the same reactions as the use of embryonic stem cells.
Bernard Siegel, the founder and director of the Regenerative Medicine Foundation and executive director of the newly formed Healthspan Action Coalition (HSAC), believes that stem cell science is rapidly approaching tipping point and changing all of medical science. (For disclosure, I do consulting work for HSAC). Siegel says that regenerative medicine has become a new pillar of medicine that has recently been fast-tracked by new technology.
Artificial intelligence is speeding up discoveries and the convergence of key disciplines, as demonstrated in Atala’s lab, which is creating complex new medical products that replace the body’s natural parts. Just as importantly, those parts are genetically matched and pose no risk of rejection.
These new technologies must be regulated, which can be a challenge, Siegel notes. “Cell therapies represent a challenge to the existing regulatory structure, including payment, reimbursement and infrastructure issues that 20 years ago, didn’t exist.” Now the FDA and other agencies are faced with this revolution, and they’re just beginning to adapt.
Siegel cited the 2021 FDA Modernization Act as a major step. The Act allows drug developers to use alternatives to animal testing in investigating the safety and efficacy of new compounds, loosening the agency’s requirement for extensive animal testing before a new drug can move into clinical trials. The Act is a recognition of the profound effect that cultured human cells are having on research. Being able to test drugs using actual human cells promises to be far safer and more accurate in predicting how they will act in the human body, and could accelerate drug development.
Siegel, a longtime veteran and founding father of several health advocacy organizations, believes this work helped bring cell therapies to people sooner rather than later. His new focus, through the HSAC, is to leverage regenerative medicine into extending not just the lifespan but the worldwide human healthspan, the period of life lived with health and vigor. “When you look at the HSAC as a tree,” asks Siegel, “what are the roots of that tree? Stem cell science and the huge ecosystem it has created.” The study of human aging is another root to the tree that has potential to lengthen healthspans.
The revolutionary science underlying the extension of the healthspan needs to be available to the whole world, Siegel says. “We need to take all these roots and come up with a way to improve the life of all mankind,” he says. “Everyone should be able to take advantage of this promising new world.”
Joy Milne's unusual sense of smell led Dr. Tilo Kunath, a neurobiologist at the Centre for Regenerative Medicine at the University of Edinburgh, and a host of other scientists, to develop a new diagnostic test for Parkinson's.
Forty years ago, Joy Milne, a nurse from Perth, Scotland, noticed a musky odor coming from her husband, Les. At first, Milne thought the smell was a result of bad hygiene and badgered her husband to take longer showers. But when the smell persisted, Milne learned to live with it, not wanting to hurt her husband's feelings.
Twelve years after she first noticed the "woodsy" smell, Les was diagnosed at the age of 44 with Parkinson's Disease, a neurodegenerative condition characterized by lack of dopamine production and loss of movement. Parkinson's Disease currently affects more than 10 million people worldwide.
Milne spent the next several years believing the strange smell was exclusive to her husband. But to her surprise, at a local support group meeting in 2012, she caught the familiar scent once again, hanging over the group like a cloud. Stunned, Milne started to wonder if the smell was the result of Parkinson's Disease itself.
Milne's discovery led her to Dr. Tilo Kunath, a neurobiologist at the Centre for Regenerative Medicine at the University of Edinburgh. Together, Milne, Kunath, and a host of other scientists would use Milne's unusual sense of smell to develop a new diagnostic test, now in development and poised to revolutionize the treatment of Parkinson's Disease.
"Joy was in the audience during a talk I was giving on my work, which has to do with Parkinson's and stem cell biology," Kunath says. "During the patient engagement portion of the talk, she asked me if Parkinson's had a smell to it." Confused, Kunath said he had never heard of this – but for months after his talk he continued to turn the question over in his mind.
Kunath knew from his research that the skin's microbiome changes during different disease processes, releasing metabolites that can give off odors. In the medical literature, diseases like melanoma and Type 2 diabetes have been known to carry a specific scent – but no such connection had been made with Parkinson's. If people could smell Parkinson's, he thought, then it stood to reason that those metabolites could be isolated, identified, and used to potentially diagnose Parkinson's by their presence alone.
First, Kunath and his colleagues decided to test Milne's sense of smell. "I got in touch with Joy again and we designed a protocol to test her sense of smell without her having to be around patients," says Kunath, which could have affected the validity of the test. In his spare time, Kunath collected t-shirt samples from people diagnosed with Parkinson's and from others without the diagnosis and gave them to Milne to smell. In 100 percent of the samples, Milne was able to detect whether a person had Parkinson's based on smell alone. Amazingly, Milne was even able to detect the "Parkinson's scent" in a shirt from the control group – someone who did not have a Parkinson's diagnosis, but would go on to be diagnosed nine months later.
From the initial study, the team discovered that Parkinson's did have a smell, that Milne – inexplicably – could detect it, and that she could detect it long before diagnosis like she had with her husband, Les. But the experiments revealed other things that the team hadn't been expecting.
"One surprising thing we learned from that experiment was that the odor was always located in the back of the shirt – never in the armpit, where we expected the smell to be," Kunath says. "I had a chance meeting with a dermatologist and he said the smell was due to the patient's sebum, which are greasy secretions that are really dense on your upper back. We have sweat glands, instead of sebum, in our armpits." Patients with Parkinson's are also known to have increased sebum production.
With the knowledge that a patient's sebum was the source of the unusual smell, researchers could go on to investigate exactly what metabolites were in the sebum and in what amounts. Kunath, along with his associate, Dr. Perdita Barran, collected and analyzed sebum samples from 64 participants across the United Kingdom. Once the samples were collected, Barran and others analyzed it using a method called gas chromatography mass spectrometry, or GS-MC, which separated, weighed and helped identify the individual compounds present in each sebum sample.
Barran's team can now correctly identify Parkinson's in nine out of 10 patients – a much quicker and more accurate way to diagnose than what clinicians do now.
"The compounds we've identified in the sebum are not unique to people with Parkinson's, but they are differently expressed," says Barran, a professor of mass spectrometry at the University of Manchester. "So this test we're developing now is not a black-and-white, do-you-have-something kind of test, but rather how much of these compounds do you have compared to other people and other compounds." The team identified over a dozen compounds that were present in the sebum of Parkinson's patients in much larger amounts than the control group.
Using only the GC-MS and a sebum swab test, Barran's team can now correctly identify Parkinson's in nine out of 10 patients – a much quicker and more accurate way to diagnose than what clinicians do now.
"At the moment, a clinical diagnosis is based on the patient's physical symptoms," Barran says, and determining whether a patient has Parkinson's is often a long and drawn-out process of elimination. "Doctors might say that a group of symptoms looks like Parkinson's, but there are other reasons people might have those symptoms, and it might take another year before they're certain," Barran says. "Some of those symptoms are just signs of aging, and other symptoms like tremor are present in recovering alcoholics or people with other kinds of dementia." People under the age of 40 with Parkinson's symptoms, who present with stiff arms, are often misdiagnosed with carpal tunnel syndrome, she adds.
Additionally, by the time physical symptoms are present, Parkinson's patients have already lost a substantial amount of dopamine receptors – about sixty percent -- in the brain's basal ganglia. Getting a diagnosis before physical symptoms appear would mean earlier interventions that could prevent dopamine loss and preserve regular movement, Barran says.
"Early diagnosis is good if it means there's a chance of early intervention," says Barran. "It stops the process of dopamine loss, which means that motor symptoms potentially will not happen, or the onset of symptoms will be substantially delayed." Barran's team is in the processing of streamlining the sebum test so that definitive results will be ready in just two minutes.
"What we're doing right now will be a very inexpensive test, a rapid-screen test, and that will encourage people to self-sample and test at home," says Barran. In addition to diagnosing Parkinson's, she says, this test could also be potentially useful to determine if medications were at a therapeutic dose in people who have the disease, since the odor is strongest in people whose symptoms are least controlled by medication.
"When symptoms are under control, the odor is lower," Barran says. "Potentially this would allow patients and clinicians to see whether their symptoms are being managed properly with medication, or perhaps if they're being overmedicated." Hypothetically, patients could also use the test to determine if interventions like diet and exercise are effective at keeping Parkinson's controlled.
"We hope within the next two to five years we will have a test available."
Barran is now running another clinical trial – one that determines whether they can diagnose at an earlier stage and whether they can identify a difference in sebum samples between different forms of Parkinson's or diseases that have Parkinson's-like symptoms, such as Lewy Body Dementia.
"Within the next one to two years, we hope to be running a trial in the Manchester area for those people who do not have motor symptoms but are at risk for developing dementia due to symptoms like loss of smell and sleep difficulty," Barran had said in 2019. "If we can establish that, we can roll out a test that determines if you have Parkinson's or not with those first pre-motor symptoms, and then at what stage. We hope within the next two to five years we will have a test available."
In a 2022 study, published in the American Chemical Society, researchers used mass spectrometry to analyze sebum from skin swabs for the presence of the specific molecules. They found that some specific molecules are present only in people who have Parkinson’s. Now they hope that the same method can be used in regular diagnostic labs. The test, many years in the making, is inching its way to the clinic.
"We would likely first give this test to people who are at risk due to a genetic predisposition, or who are at risk based on prodomal symptoms, like people who suffer from a REM sleep disorder who have a 50 to 70 percent chance of developing Parkinson's within a ten year period," Barran says. "Those would be people who would benefit from early therapeutic intervention. For the normal population, it isn't beneficial at the moment to know until we have therapeutic interventions that can be useful."
Milne's husband, Les, passed away from complications of Parkinson's Disease in 2015. But thanks to him and the dedication of his wife, Joy, science may have found a way to someday prolong the lives of others with this devastating disease. Sometimes she can smell people who have Parkinson’s while in the supermarket or walking down the street but has been told by medical ethicists she cannot tell them, Milne said in an interview with the Guardian. But once the test becomes available in the clinics, it will do the job for her.
[Ed. Note: A older version of this hit article originally ran on September 3, 2019.]