Beyond Henrietta Lacks: How the Law Has Denied Every American Ownership Rights to Their Own Cells
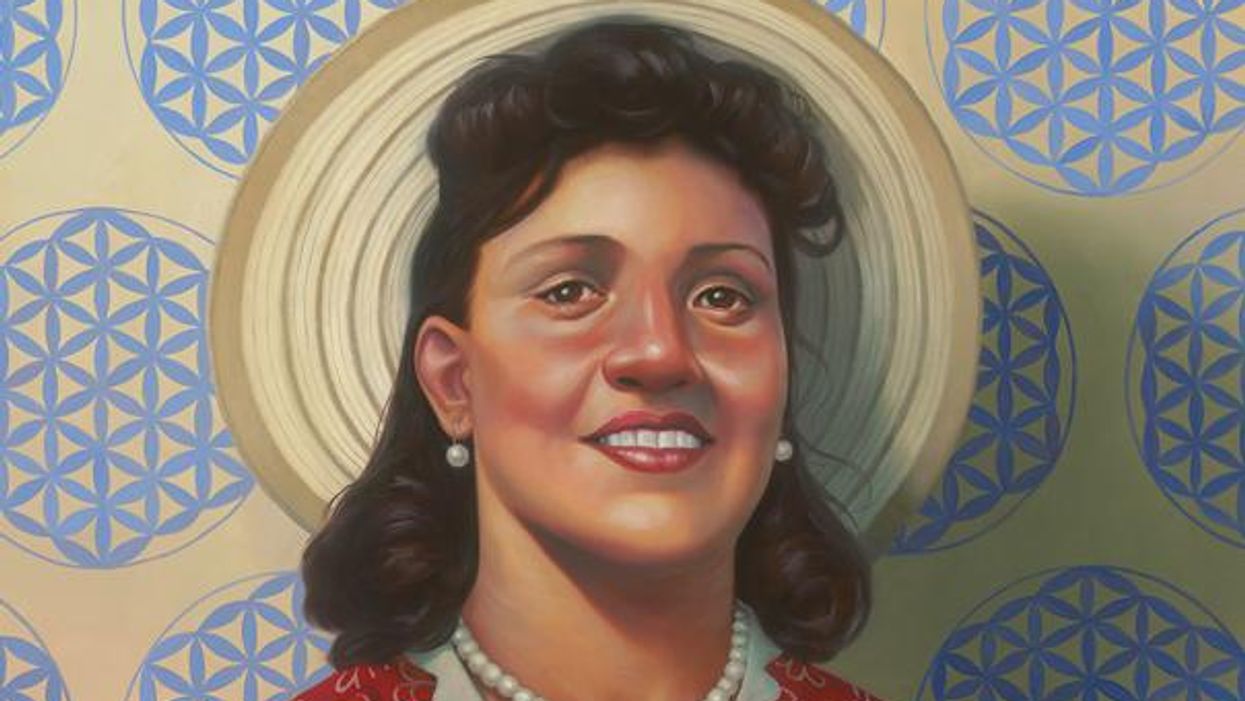
A 2017 portrait of Henrietta Lacks.
The common perception is that Henrietta Lacks was a victim of poverty and racism when in 1951 doctors took samples of her cervical cancer without her knowledge or permission and turned them into the world's first immortalized cell line, which they called HeLa. The cell line became a workhorse of biomedical research and facilitated the creation of medical treatments and cures worth untold billions of dollars. Neither Lacks nor her family ever received a penny of those riches.
But racism and poverty is not to blame for Lacks' exploitation—the reality is even worse. In fact all patients, then and now, regardless of social or economic status, have absolutely no right to cells that are taken from their bodies. Some have called this biological slavery.
How We Got Here
The case that established this legal precedent is Moore v. Regents of the University of California.
John Moore was diagnosed with hairy-cell leukemia in 1976 and his spleen was removed as part of standard treatment at the UCLA Medical Center. On initial examination his physician, David W. Golde, had discovered some unusual qualities to Moore's cells and made plans prior to the surgery to have the tissue saved for research rather than discarded as waste. That research began almost immediately.
"On both sides of the case, legal experts and cultural observers cautioned that ownership of a human body was the first step on the slippery slope to 'bioslavery.'"
Even after Moore moved to Seattle, Golde kept bringing him back to Los Angeles to collect additional samples of blood and tissue, saying it was part of his treatment. When Moore asked if the work could be done in Seattle, he was told no. Golde's charade even went so far as claiming to find a low-income subsidy to pay for Moore's flights and put him up in a ritzy hotel to get him to return to Los Angeles, while paying for those out of his own pocket.
Moore became suspicious when he was asked to sign new consent forms giving up all rights to his biological samples and he hired an attorney to look into the matter. It turned out that Golde had been lying to his patient all along; he had been collecting samples unnecessary to Moore's treatment and had turned them into a cell line that he and UCLA had patented and already collected millions of dollars in compensation. The market for the cell lines was estimated at $3 billion by 1990.
Moore felt he had been taken advantage of and filed suit to claim a share of the money that had been made off of his body. "On both sides of the case, legal experts and cultural observers cautioned that ownership of a human body was the first step on the slippery slope to 'bioslavery,'" wrote Priscilla Wald, a professor at Duke University whose career has focused on issues of medicine and culture. "Moore could be viewed as asking to commodify his own body part or be seen as the victim of the theft of his most private and inalienable information."
The case bounced around different levels of the court system with conflicting verdicts for nearly six years until the California Supreme Court ruled on July 9, 1990 that Moore had no legal rights to cells and tissue once they were removed from his body.
The court made a utilitarian argument that the cells had no value until scientists manipulated them in the lab. And it would be too burdensome for researchers to track individual donations and subsequent cell lines to assure that they had been ethically gathered and used. It would impinge on the free sharing of materials between scientists, slow research, and harm the public good that arose from such research.
"In effect, what Moore is asking us to do is impose a tort duty on scientists to investigate the consensual pedigree of each human cell sample used in research," the majority wrote. In other words, researchers don't need to ask any questions about the materials they are using.
One member of the court did not see it that way. In his dissent, Stanley Mosk raised the specter of slavery that "arises wherever scientists or industrialists claim, as defendants have here, the right to appropriate and exploit a patient's tissue for their sole economic benefit—the right, in other words, to freely mine or harvest valuable physical properties of the patient's body. … This is particularly true when, as here, the parties are not in equal bargaining positions."
Mosk also cited the appeals court decision that the majority overturned: "If this science has become for profit, then we fail to see any justification for excluding the patient from participation in those profits."
But the majority bought the arguments that Golde, UCLA, and the nascent biotechnology industry in California had made in amici briefs filed throughout the legal proceedings. The road was now cleared for them to develop products worth billions without having to worry about or share with the persons who provided the raw materials upon which their research was based.
Critical Views
Biomedical research requires a continuous and ever-growing supply of human materials for the foundation of its ongoing work. If an increasing number of patients come to feel as John Moore did, that the system is ripping them off, then they become much less likely to consent to use of their materials in future research.
Some legal and ethical scholars say that donors should be able to limit the types of research allowed for their tissues and researchers should be monitored to assure compliance with those agreements. For example, today it is commonplace for companies to certify that their clothing is not made by child labor, their coffee is grown under fair trade conditions, that food labeled kosher is properly handled. Should we ask any less of our pharmaceuticals than that the donors whose cells made such products possible have been treated honestly and fairly, and share in the financial bounty that comes from such drugs?
Protection of individual rights is a hallmark of the American legal system, says Lisa Ikemoto, a law professor at the University of California Davis. "Putting the needs of a generalized public over the interests of a few often rests on devaluation of the humanity of the few," she writes in a reimagined version of the Moore decision that upholds Moore's property claims to his excised cells. The commentary is in a chapter of a forthcoming book in the Feminist Judgment series, where authors may only use legal precedent in effect at the time of the original decision.
"Why is the law willing to confer property rights upon some while denying the same rights to others?" asks Radhika Rao, a professor at the University of California, Hastings College of the Law. "The researchers who invest intellectual capital and the companies and universities that invest financial capital are permitted to reap profits from human research, so why not those who provide the human capital in the form of their own bodies?" It might be seen as a kind of sweat equity where cash strapped patients make a valuable in kind contribution to the enterprise.
The Moore court also made a big deal about inhibiting the free exchange of samples between scientists. That has become much less the situation over the more than three decades since the decision was handed down. Ironically, this decision, as well as other laws and regulations, have since strengthened the power of patents in biomedicine and by doing so have increased secrecy and limited sharing.
"Although the research community theoretically endorses the sharing of research, in reality sharing is commonly compromised by the aggressive pursuit and defense of patents and by the use of licensing fees that hinder collaboration and development," Robert D. Truog, Harvard Medical School ethicist and colleagues wrote in 2012 in the journal Science. "We believe that measures are required to ensure that patients not bear all of the altruistic burden of promoting medical research."
Additionally, the increased complexity of research and the need for exacting standardization of materials has given rise to an industry that supplies certified chemical reagents, cell lines, and whole animals bred to have specific genetic traits to meet research needs. This has been more efficient for research and has helped to ensure that results from one lab can be reproduced in another.
The Court's rationale of fostering collaboration and free exchange of materials between researchers also has been undercut by the changing structure of that research. Big pharma has shrunk the size of its own research labs and over the last decade has worked out cooperative agreements with major research universities where the companies contribute to the research budget and in return have first dibs on any findings (and sometimes a share of patent rights) that come out of those university labs. It has had a chilling effect on the exchange of materials between universities.
Perhaps tracking cell line donors and use restrictions on those donations might have been burdensome to researchers when Moore was being litigated. Some labs probably still kept their cell line records on 3x5 index cards, computers were primarily expensive room-size behemoths with limited capacity, the internet barely existed, and there was no cloud storage.
But that was the dawn of a new technological age and standards have changed. Now cell lines are kept in state-of-the-art sub zero storage units, tagged with the source, type of tissue, date gathered and often other information. Adding a few more data fields and contacting the donor if and when appropriate does not seem likely to disrupt the research process, as the court asserted.
Forging the Future
"U.S. universities are awarded almost 3,000 patents each year. They earn more than $2 billion each year from patent royalties. Sharing a modest portion of these profits is a novel method for creating a greater sense of fairness in research relationships that we think is worth exploring," wrote Mark Yarborough, a bioethicist at the University of California Davis Medical School, and colleagues. That was penned nearly a decade ago and those numbers have only grown.
The Michigan BioTrust for Health might serve as a useful model in tackling some of these issues. Dried blood spots have been collected from all newborns for half a century to be tested for certain genetic diseases, but controversy arose when the huge archive of dried spots was used for other research projects. As a result, the state created a nonprofit organization to in essence become a biobank and manage access to these spots only for specific purposes, and also to share any revenue that might arise from that research.
"If there can be no property in a whole living person, does it stand to reason that there can be no property in any part of a living person? If there were, can it be said that this could equate to some sort of 'biological slavery'?" Irish ethicist Asim A. Sheikh wrote several years ago. "Any amount of effort spent pondering the issue of 'ownership' in human biological materials with existing law leaves more questions than answers."
Perhaps the biggest question will arise when -- not if but when -- it becomes possible to clone a human being. Would a human clone be a legal person or the property of those who created it? Current legal precedent points to it being the latter.
Today, October 4, is the 70th anniversary of Henrietta Lacks' death from cancer. Over those decades her immortalized cells have helped make possible miraculous advances in medicine and have had a role in generating billions of dollars in profits. Surviving family members have spoken many times about seeking a share of those profits in the name of social justice; they intend to file lawsuits today. Such cases will succeed or fail on their own merits. But regardless of their specific outcomes, one can hope that they spark a larger public discussion of the role of patients in the biomedical research enterprise and lead to establishing a legal and financial claim for their contributions toward the next generation of biomedical research.
DNA- and RNA-based electronic implants may revolutionize healthcare
The test tubes contain tiny DNA/enzyme-based circuits, which comprise TRUMPET, a new type of electronic device, smaller than a cell.
Implantable electronic devices can significantly improve patients’ quality of life. A pacemaker can encourage the heart to beat more regularly. A neural implant, usually placed at the back of the skull, can help brain function and encourage higher neural activity. Current research on neural implants finds them helpful to patients with Parkinson’s disease, vision loss, hearing loss, and other nerve damage problems. Several of these implants, such as Elon Musk’s Neuralink, have already been approved by the FDA for human use.
Yet, pacemakers, neural implants, and other such electronic devices are not without problems. They require constant electricity, limited through batteries that need replacements. They also cause scarring. “The problem with doing this with electronics is that scar tissue forms,” explains Kate Adamala, an assistant professor of cell biology at the University of Minnesota Twin Cities. “Anytime you have something hard interacting with something soft [like muscle, skin, or tissue], the soft thing will scar. That's why there are no long-term neural implants right now.” To overcome these challenges, scientists are turning to biocomputing processes that use organic materials like DNA and RNA. Other promised benefits include “diagnostics and possibly therapeutic action, operating as nanorobots in living organisms,” writes Evgeny Katz, a professor of bioelectronics at Clarkson University, in his book DNA- And RNA-Based Computing Systems.
While a computer gives these inputs in binary code or "bits," such as a 0 or 1, biocomputing uses DNA strands as inputs, whether double or single-stranded, and often uses fluorescent RNA as an output.
Adamala’s research focuses on developing such biocomputing systems using DNA, RNA, proteins, and lipids. Using these molecules in the biocomputing systems allows the latter to be biocompatible with the human body, resulting in a natural healing process. In a recent Nature Communications study, Adamala and her team created a new biocomputing platform called TRUMPET (Transcriptional RNA Universal Multi-Purpose GatE PlaTform) which acts like a DNA-powered computer chip. “These biological systems can heal if you design them correctly,” adds Adamala. “So you can imagine a computer that will eventually heal itself.”
The basics of biocomputing
Biocomputing and regular computing have many similarities. Like regular computing, biocomputing works by running information through a series of gates, usually logic gates. A logic gate works as a fork in the road for an electronic circuit. The input will travel one way or another, giving two different outputs. An example logic gate is the AND gate, which has two inputs (A and B) and two different results. If both A and B are 1, the AND gate output will be 1. If only A is 1 and B is 0, the output will be 0 and vice versa. If both A and B are 0, the result will be 0. While a computer gives these inputs in binary code or "bits," such as a 0 or 1, biocomputing uses DNA strands as inputs, whether double or single-stranded, and often uses fluorescent RNA as an output. In this case, the DNA enters the logic gate as a single or double strand.
If the DNA is double-stranded, the system “digests” the DNA or destroys it, which results in non-fluorescence or “0” output. Conversely, if the DNA is single-stranded, it won’t be digested and instead will be copied by several enzymes in the biocomputing system, resulting in fluorescent RNA or a “1” output. And the output for this type of binary system can be expanded beyond fluorescence or not. For example, a “1” output might be the production of the enzyme insulin, while a “0” may be that no insulin is produced. “This kind of synergy between biology and computation is the essence of biocomputing,” says Stephanie Forrest, a professor and the director of the Biodesign Center for Biocomputing, Security and Society at Arizona State University.
Biocomputing circles are made of DNA, RNA, proteins and even bacteria.
Evgeny Katz
The TRUMPET’s promise
Depending on whether the biocomputing system is placed directly inside a cell within the human body, or run in a test-tube, different environmental factors play a role. When an output is produced inside a cell, the cell's natural processes can amplify this output (for example, a specific protein or DNA strand), creating a solid signal. However, these cells can also be very leaky. “You want the cells to do the thing you ask them to do before they finish whatever their businesses, which is to grow, replicate, metabolize,” Adamala explains. “However, often the gate may be triggered without the right inputs, creating a false positive signal. So that's why natural logic gates are often leaky." While biocomputing outside a cell in a test tube can allow for tighter control over the logic gates, the outputs or signals cannot be amplified by a cell and are less potent.
TRUMPET, which is smaller than a cell, taps into both cellular and non-cellular biocomputing benefits. “At its core, it is a nonliving logic gate system,” Adamala states, “It's a DNA-based logic gate system. But because we use enzymes, and the readout is enzymatic [where an enzyme replicates the fluorescent RNA], we end up with signal amplification." This readout means that the output from the TRUMPET system, a fluorescent RNA strand, can be replicated by nearby enzymes in the platform, making the light signal stronger. "So it combines the best of both worlds,” Adamala adds.
These organic-based systems could detect cancer cells or low insulin levels inside a patient’s body.
The TRUMPET biocomputing process is relatively straightforward. “If the DNA [input] shows up as single-stranded, it will not be digested [by the logic gate], and you get this nice fluorescent output as the RNA is made from the single-stranded DNA, and that's a 1,” Adamala explains. "And if the DNA input is double-stranded, it gets digested by the enzymes in the logic gate, and there is no RNA created from the DNA, so there is no fluorescence, and the output is 0." On the story's leading image above, if the tube is "lit" with a purple color, that is a binary 1 signal for computing. If it's "off" it is a 0.
While still in research, TRUMPET and other biocomputing systems promise significant benefits to personalized healthcare and medicine. These organic-based systems could detect cancer cells or low insulin levels inside a patient’s body. The study’s lead author and graduate student Judee Sharon is already beginning to research TRUMPET's ability for earlier cancer diagnoses. Because the inputs for TRUMPET are single or double-stranded DNA, any mutated or cancerous DNA could theoretically be detected from the platform through the biocomputing process. Theoretically, devices like TRUMPET could be used to detect cancer and other diseases earlier.
Adamala sees TRUMPET not only as a detection system but also as a potential cancer drug delivery system. “Ideally, you would like the drug only to turn on when it senses the presence of a cancer cell. And that's how we use the logic gates, which work in response to inputs like cancerous DNA. Then the output can be the production of a small molecule or the release of a small molecule that can then go and kill what needs killing, in this case, a cancer cell. So we would like to develop applications that use this technology to control the logic gate response of a drug’s delivery to a cell.”
Although platforms like TRUMPET are making progress, a lot more work must be done before they can be used commercially. “The process of translating mechanisms and architecture from biology to computing and vice versa is still an art rather than a science,” says Forrest. “It requires deep computer science and biology knowledge,” she adds. “Some people have compared interdisciplinary science to fusion restaurants—not all combinations are successful, but when they are, the results are remarkable.”
Crickets are low on fat, high on protein, and can be farmed sustainably. They are also crunchy.
In today’s podcast episode, Leaps.org Deputy Editor Lina Zeldovich speaks about the health and ecological benefits of farming crickets for human consumption with Bicky Nguyen, who joins Lina from Vietnam. Bicky and her business partner Nam Dang operate an insect farm named CricketOne. Motivated by the idea of sustainable and healthy protein production, they started their unconventional endeavor a few years ago, despite numerous naysayers who didn’t believe that humans would ever consider munching on bugs.
Yet, making creepy crawlers part of our diet offers many health and planetary advantages. Food production needs to match the rise in global population, estimated to reach 10 billion by 2050. One challenge is that some of our current practices are inefficient, polluting and wasteful. According to nonprofit EarthSave.org, it takes 2,500 gallons of water, 12 pounds of grain, 35 pounds of topsoil and the energy equivalent of one gallon of gasoline to produce one pound of feedlot beef, although exact statistics vary between sources.
Meanwhile, insects are easy to grow, high on protein and low on fat. When roasted with salt, they make crunchy snacks. When chopped up, they transform into delicious pâtes, says Bicky, who invents her own cricket recipes and serves them at industry and public events. Maybe that’s why some research predicts that edible insects market may grow to almost $10 billion by 2030. Tune in for a delectable chat on this alternative and sustainable protein.
Listen on Apple | Listen on Spotify | Listen on Stitcher | Listen on Amazon | Listen on Google
Further reading:
More info on Bicky Nguyen
https://yseali.fulbright.edu.vn/en/faculty/bicky-n...
The environmental footprint of beef production
https://www.earthsave.org/environment.htm
https://www.watercalculator.org/news/articles/beef-king-big-water-footprints/
https://www.frontiersin.org/articles/10.3389/fsufs.2019.00005/full
https://ourworldindata.org/carbon-footprint-food-methane
Insect farming as a source of sustainable protein
https://www.insectgourmet.com/insect-farming-growing-bugs-for-protein/
https://www.sciencedirect.com/topics/agricultural-and-biological-sciences/insect-farming
Cricket flour is taking the world by storm
https://www.cricketflours.com/
https://talk-commerce.com/blog/what-brands-use-cricket-flour-and-why/
Lina Zeldovich has written about science, medicine and technology for Popular Science, Smithsonian, National Geographic, Scientific American, Reader’s Digest, the New York Times and other major national and international publications. A Columbia J-School alumna, she has won several awards for her stories, including the ASJA Crisis Coverage Award for Covid reporting, and has been a contributing editor at Nautilus Magazine. In 2021, Zeldovich released her first book, The Other Dark Matter, published by the University of Chicago Press, about the science and business of turning waste into wealth and health. You can find her on http://linazeldovich.com/ and @linazeldovich.