Study Shows “Living Drug” Can Provide a Lasting Cure for Cancer
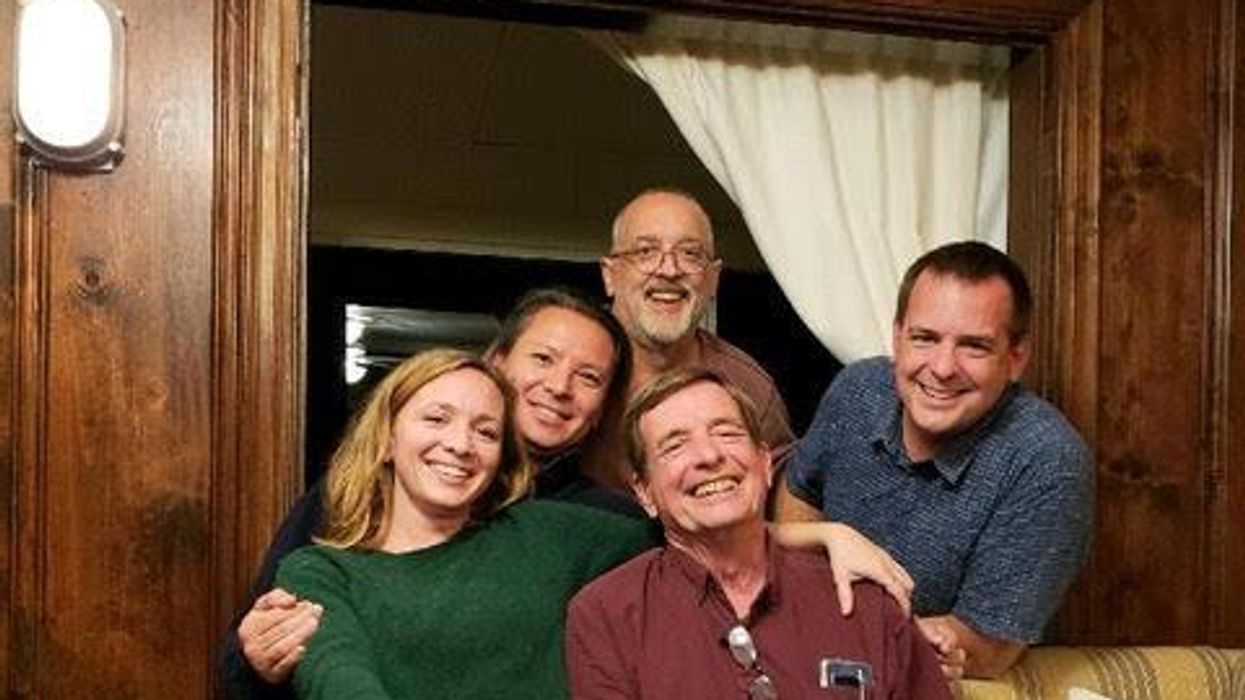
A recent study by researchers at the University of Pennsylvania examined how CAR-T therapy helped Doug Olson beat a cancer death sentence for over a decade - and how it could work for more people.
Doug Olson was 49 when he was diagnosed with chronic lymphocytic leukemia, a blood cancer that strikes 21,000 Americans annually. Although the disease kills most patients within a decade, Olson’s case progressed more slowly, and courses of mild chemotherapy kept him healthy for 13 years. Then, when he was 62, the medication stopped working. The cancer had mutated, his doctor explained, becoming resistant to standard remedies. Harsher forms of chemo might buy him a few months, but their side effects would be debilitating. It was time to consider the treatment of last resort: a bone-marrow transplant.
Olson, a scientist who developed blood-testing instruments, knew the odds. There was only a 50 percent chance that a transplant would cure him. There was a 20 percent chance that the agonizing procedure—which involves destroying the patient’s marrow with chemo and radiation, then infusing his blood with donated stem cells—would kill him. If he survived, he would face the danger of graft-versus-host disease, in which the donor’s cells attack the recipient’s tissues. To prevent it, he would have to take immunosuppressant drugs, increasing the risk of infections. He could end up with pneumonia if one of his three grandchildren caught a sniffle. “I was being pushed into a corner,” Olson recalls, “with very little room to move.”
Soon afterward, however, his doctor revealed a possible escape route. He and some colleagues at the University of Pennsylvania’s Abramson Cancer Center were starting a clinical trial, he said, and Olson—still mostly symptom-free—might be a good candidate. The experimental treatment, known as CAR-T therapy, would use genetic engineering to turn his T lymphocytes (immune cells that guard against viruses and other pathogens) into a weapon against cancer.
In September 2010, technicians took some of Olson’s T cells to a laboratory, where they were programmed with new molecular marching orders and coaxed to multiply into an army of millions. When they were ready, a nurse inserted a catheter into his neck. At the turn of a valve, his soldiers returned home, ready to do battle.
“I felt like I’d won the lottery,” Olson says. But he was only the second person in the world to receive this “living drug,” as the University of Pennsylvania investigators called it. No one knew how long his remission would last.
Three weeks later, Olson was slammed with a 102-degree fever, nausea, and chills. The treatment had triggered two dangerous complications: cytokine release syndrome, in which immune chemicals inflame the patient’s tissues, and tumor lysis syndrome, in which toxins from dying cancer cells overwhelm the kidneys. But the crisis passed quickly, and the CAR-T cells fought on. A month after the infusion, the doctor delivered astounding news: “We can’t find any cancer in your body.”
“I felt like I’d won the lottery,” Olson says. But he was only the second person in the world to receive this “living drug,” as the University of Pennsylvania investigators called it. No one knew how long his remission would last.
An Unexpected Cure
In February 2022, the same cancer researchers reported a remarkable milestone: the trial’s first two patients had survived for more than a decade. Although Olson’s predecessor—a retired corrections officer named Bill Ludwig—died of COVID-19 complications in early 2021, both men had remained cancer-free. And the modified immune cells continued to patrol their territory, ready to kill suspected tumor cells the moment they arose.
“We can now conclude that CAR-T cells can actually cure patients with leukemia,” University of Pennsylvania immunologist Carl June, who spearheaded the development of the technique, told reporters. “We thought the cells would be gone in a month or two. The fact that they’ve survived 10 years is a major surprise.”
Even before the announcement, it was clear that CAR-T therapy could win a lasting reprieve for many patients with cancers that were once a death sentence. Since the Food and Drug Administration approved June’s version (marketed as Kymriah) in 2017, the agency has greenlighted five more such treatments for various types of leukemia, lymphoma, and myeloma. “Every single day, I take care of patients who would previously have been told they had no options,” says Rayne Rouce, a pediatric hematologist/oncologist at Texas Children’s Cancer Center. “Now we not only have a treatment option for those patients, but one that could potentially be the last therapy for their cancer that they’ll ever have to receive.”
Immunologist Carl June, middle, spearheaded development of the CAR-T therapy that gave patients Bill Ludwig, left, and Doug Olson, right, a lengthy reprieve on their terminal cancer diagnoses.
Penn Medicine
Yet the CAR-T approach doesn’t help everyone. So far, it has only shown success for blood cancers—and for those, the overall remission rate is 30 to 40 percent. “When it works, it works extraordinarily well,” says Olson’s former doctor, David Porter, director of Penn’s blood and bone marrow transplant program. “It’s important to know why it works, but it’s equally important to know why it doesn’t—and how we can fix that.”
The team’s study, published in the journal Nature, offers a wealth of data on what worked for these two patients. It may also hold clues for how to make the therapy effective for more people.
Building a Better T Cell
Carl June didn’t set out to cure cancer, but his serendipitous career path—and a personal tragedy—helped him achieve insights that had eluded other researchers. In 1971, hoping to avoid combat in Vietnam, he applied to the U.S. Naval Academy in Annapolis, Maryland. June showed a knack for biology, so the Navy sent him on to Baylor College of Medicine. He fell in love with immunology during a fellowship researching malaria vaccines in Switzerland. Later, the Navy deployed him to the Fred Hutchinson Cancer Research Center in Seattle to study bone marrow transplantation.
There, June became part of the first research team to learn how to culture T cells efficiently in a lab. After moving on to the National Naval Medical Center in the ’80s, he used that knowledge to combat the newly emerging AIDS epidemic. HIV, the virus that causes the disease, invades T cells and eventually destroys them. June and his post-doc Bruce Levine developed a method to restore patients’ depleted cell populations, using tiny magnetic beads to deliver growth-stimulating proteins. Infused into the body, the new T cells effectively boosted immune function.
In 1999, after leaving the Navy, June joined the University of Pennsylvania. His wife, who’d been diagnosed with ovarian cancer, died two years later, leaving three young children. “I had not known what it was like to be on the other side of the bed,” he recalls. Watching her suffer through grueling but futile chemotherapy, followed by an unsuccessful bone-marrow transplant, he resolved to focus on finding better cancer treatments. He started with leukemia—a family of diseases in which mutant white blood cells proliferate in the marrow.
Cancer is highly skilled at slipping through the immune system’s defenses. T cells, for example, detect pathogens by latching onto them with receptors designed to recognize foreign proteins. Leukemia cells evade detection, in part, by masquerading as normal white blood cells—that is, as part of the immune system itself.
June planned to use a viral vector no one had tried before: HIV.
To June, chimeric antigen receptor (CAR) T cells looked like a promising tool for unmasking and destroying the impostors. Developed in the early ’90s, these cells could be programmed to identify a target protein, and to kill any pathogen that displayed it. To do the programming, you spliced together snippets of DNA and inserted them into a disabled virus. Next, you removed some of the patient’s T cells and infected them with the virus, which genetically hijacked its new hosts—instructing them to find and slay the patient’s particular type of cancer cells. When the T cells multiplied, their descendants carried the new genetic code. You then infused those modified cells into the patient, where they went to war against their designated enemy.
Or that’s what happened in theory. Many scientists had tried to develop therapies using CAR-T cells, but none had succeeded. Although the technique worked in lab animals, the cells either died out or lost their potency in humans.
But June had the advantage of his years nurturing T cells for AIDS patients, as well as the technology he’d developed with Levine (who’d followed him to Penn with other team members). He also planned to use a viral vector no one had tried before: HIV, which had evolved to thrive in human T cells and could be altered to avoid causing disease. By the summer of 2010, he was ready to test CAR-T therapy against chronic lymphocytic leukemia (CLL), the most common form of the disease in adults.
Three patients signed up for the trial, including Doug Olson and Bill Ludwig. A portion of each man’s T cells were reprogrammed to detect a protein found only on B lymphocytes, the type of white blood cells affected by CLL. Their genetic instructions ordered them to destroy any cell carrying the protein, known as CD19, and to multiply whenever they encountered one. This meant the patients would forfeit all their B cells, not just cancerous ones—but regular injections of gamma globulins (a cocktail of antibodies) would make up for the loss.
After being infused with the CAR-T cells, all three men suffered high fevers and potentially life-threatening inflammation, but all pulled through without lasting damage. The third patient experienced a partial remission and survived for eight months. Olson and Ludwig were cured.
Learning What Works
Since those first infusions, researchers have developed reliable ways to prevent or treat the side effects of CAR-T therapy, greatly reducing its risks. They’ve also been experimenting with combination therapies—pairing CAR-T with chemo, cancer vaccines, and immunotherapy drugs called checkpoint inhibitors—to improve its success rate. But CAR-T cells are still ineffective for at least 60 percent of blood cancer patients. And they remain in the experimental stage for solid tumors (including pancreatic cancer, mesothelioma, and glioblastoma), whose greater complexity make them harder to attack.
The new Nature study offers clues that could fuel further advances. The Penn team “profiled these cells at a level where we can almost say, ‘These are the characteristics that a T cell would need to survive 10 years,’” says Rouce, the physician at Texas Children’s Cancer Center.
One surprising finding involves how CAR-T cells change in the body over time. At first, those that Olson and Ludwig received showed the hallmarks of “killer” T-cells (also known as CD8 cells)—highly active lymphocytes bent on exterminating every tumor cell in sight. After several months, however, the population shifted toward “helper” T-cells (or CD4s), which aid in forming long-term immune memory but are normally incapable of direct aggression. Over the years, the numbers swung back and forth, until only helper cells remained. Those cells showed markers suggesting they were too exhausted to function—but in the lab, they were able not only to recognize but to destroy cancer cells.
June and his team suspect that those tired-looking helper cells had enough oomph to kill off any B cells Olson and Ludwig made, keeping the pair’s cancers permanently at bay. If so, that could prompt new approaches to selecting cells for CAR-T therapy. Maybe starting with a mix of cell types—not only CD8s, but CD4s and other varieties—would work better than using CD8s alone. Or perhaps inducing changes in cell populations at different times would help.
Another potential avenue for improvement is starting with healthier cells. Evidence from this and other trials hints that patients whose T cells are more robust to begin with respond better when their cells are used in CAR-T therapy. The Penn team recently completed a clinical trial in which CLL patients were treated with ibrutinib—a drug that enhances T-cell function—before their CAR-T cells were manufactured. The response rate, says David Porter, was “very high,” with most patients remaining cancer-free a year after being infused with the souped-up cells.
Such approaches, he adds, are essential to achieving the next phase in CAR-T therapy: “Getting it to work not just in more people, but in everybody.”
Doug Olson enjoys nature - and having a future.
Penn Medicine
To grasp what that could mean, it helps to talk with Doug Olson, who’s now 75. In the years since his infusion, he has watched his four children forge careers, and his grandkids reach their teens. He has built a business and enjoyed the rewards of semi-retirement. He’s done volunteer and advocacy work for cancer patients, run half-marathons, sailed the Caribbean, and ridden his bike along the sun-dappled roads of Silicon Valley, his current home.
And in his spare moments, he has just sat there feeling grateful. “You don’t really appreciate the effect of having a lethal disease until it’s not there anymore,” he says. “The world looks different when you have a future.”
This article was first published on Leaps.org on March 24, 2022.
An implant, combined with the glasses and tiny video camera modeled in this photo, could improve the eyesight of millions of people with degenerative eye diseases in the coming years.
For millions of people with macular degeneration, treatment options are slim. The disease causes loss of central vision, which allows us to see straight ahead, and is highly dependent on age, with people over 75 at approximately 30% risk of developing the disorder. The BrightFocus Foundation estimates 11 million people in the U.S. currently have one of three forms of the disease.
Recently, ophthalmologists including Daniel Palanker at Stanford University published research showing advances in the PRIMA retinal implant, which could help people with advanced, age-related macular degeneration regain some of their sight. In a feasibility study, five patients had a pixelated chip implanted behind the retina, and three were able to see using their remaining peripheral vision and—thanks to the implant—their partially restored central vision at the same time.
Should people with macular degeneration be excited about these results?
“Every week, if not every day, patients come to me with this question because it's devastating when they lose their central vision,” says retinal surgeon Lynn Huang. About 40% of her patients have macular degeneration. Huang tells them that these implants, along with new medications and stem cell therapies, could be useful in the coming years.
“The goal here is to replace the missing photoreceptors with photovoltaic pixels, basically like little solar panels,” Palanker says.
That implant, a pixelated chip, works together with a tiny video camera on a specially designed pair of eyeglasses, which can be adjusted for each patient’s prescription. The video camera relays processed images to the chip, which electrically stimulates inner retinal neurons. These neurons, in turn, relay information to the brain’s visual cortex through the optic nerve. The chip restores patients’ central sight, but not completely. The artificial vision is basically monochromatic (whitish-yellowish) and fairly blurry; patients were still legally blind even after the implant, except when using a zoom function on the camera, but those with proper chip placement could make out large letters.
“The goal here is to replace the missing photoreceptors with photovoltaic pixels, basically like little solar panels,” Palanker says. These pixels, located on the implanted chip, convert light into pulsed electrical currents that stimulate retinal neurons. In time, Palanker hopes to improve the chips, resulting in bigger boosts to visual acuity.
The pixelated chips are surgically implanted during a process Palanker admits is still “a surgical learning curve.” In the study, three chips were implanted correctly, one was placed incorrectly, and another patient’s chip moved after the procedure; he did not follow post-surgical recommendations. One patient passed away during the study for unrelated reasons.
University of Maryland retinal specialist Kenneth Taubenslag, who was not involved in the study, said that subretinal surgeries have become less common in recent years, but expects implants to spur improvements in these techniques. “I think as people get more experience, [they’ll] probably get more reliable placement of the implant,” he said, pointing out that even the patient with the misplaced chip was able to gain some light perception, if not the same visual acuity as other patients.
Retinal implants have come under scrutiny lately. IEEE Spectrum reported that Second Sight, manufacturer of the Argus II implant used for people with retinitis pigmentosa, a genetic disease that causes vision loss, would no longer support the product. After selling hundreds of the implants at $150,000 apiece, company leaders announced they’d “decided to pursue an orderly wind down” of Second Sight in March 2020 in the wake of financial issues. Last month, the company announced a merger, shifting its focus to a new retinal implant, raising questions for patients who have Argus II implants.
Retinal surgeon Eugene de Juan of the University of California, San Francisco, was involved with early studies of the Argus implants, though his participation ended over a decade ago, before the device was marketed by Second Sight. He says he would consider recommending future implants to patients with macular degeneration, given the promise of the technology and the lack of other alternatives.
“I tell my patients that this is an area of active research and development, and it's getting better and better, so let's not give up hope,” de Juan says. He believes cautious optimism for Palanker’s implant is appropriate: “It's not the first, it's not the only, but it's a good approach with a good team.”
How dozens of men across Alaska (and their dogs) teamed up to save one town from a deadly outbreak
In 1925, health officials in Alaska came up with a creative solution to save a remote fishing town from a deadly disease outbreak.
During the winter of 1924, Curtis Welch – the only doctor in Nome, a remote fishing town in northwest Alaska – started noticing something strange. More and more, the children of Nome were coming to his office with sore throats.
Initially, Welch dismissed the cases as tonsillitis or some run-of-the-mill virus – but when more kids started getting sick, with some even dying, he grew alarmed. It wasn’t until early 1925, after a three-year-old boy died just two weeks after becoming ill, that Welch realized that his worst suspicions were true. The boy – and dozens of other children in town – were infected with diphtheria.
A DEADLY BACTERIA
Diphtheria is nearly nonexistent and almost unheard of in industrialized countries today. But less than a century ago, diphtheria was a household name – one that struck fear in the heart of every parent, as it was extremely contagious and particularly deadly for children.
Diphtheria – a bacterial infection – is an ugly disease. When it strikes, the bacteria eats away at the healthy tissues in a patient’s respiratory tract, leaving behind a thick, gray membrane of dead tissue that covers the patient's nose, throat, and tonsils. Not only does this membrane make it very difficult for the patient to breathe and swallow, but as the bacteria spreads through the bloodstream, it causes serious harm to the heart and kidneys. It sometimes also results in nerve damage and paralysis. Even with treatment, diphtheria kills around 10 percent of people it infects. Young children, as well as adults over the age of 60, are especially at risk.
Welch didn’t suspect diphtheria at first. He knew the illness was incredibly contagious and reasoned that many more people would be sick – specifically, the family members of the children who had died – if there truly was an outbreak. Nevertheless, the symptoms, along with the growing number of deaths, were unmistakable. By 1925 Welch knew for certain that diphtheria had come to Nome.
In desperation, Welch tried treating an infected seven-year-old girl with some expired antitoxin – but she died just a few hours after he administered it.
AN INACCESSIBLE CURE
A vaccine for diphtheria wouldn’t be widely available until the mid-1930s and early 1940s – so an outbreak of the disease meant that each of the 10,000 inhabitants of Nome were all at serious risk.
One option was to use something called an antitoxin – a serum consisting of anti-diphtheria antibodies – to treat the patients. However, the town’s reserve of diphtheria antitoxin had expired. Welch had ordered a replacement shipment of antitoxin the previous summer – but the shipping port that was set to deliver the serum had been closed due to ice, and no new antitoxin would arrive before spring of 1925. In desperation, Welch tried treating an infected seven-year-old girl with some expired antitoxin – but she died just a few hours after he administered it.
Welch radioed for help to all the major towns in Alaska as well as the US Public Health Service in Washington, DC. His telegram read: An outbreak of diphtheria is almost inevitable here. I am in urgent need of one million units of diphtheria antitoxin. Mail is the only form of transportation.
FOUR-LEGGED HEROES
When the Alaskan Board of Health learned about the outbreak, the men rushed to devise a plan to get antitoxin to Nome. Dropping the serum in by airplane was impossible, as the available planes were unsuitable for flying during Alaska’s severe winter weather, where temperatures were routinely as cold as -50 degrees Fahrenheit.
In late January 1925, roughly 30,000 units of antitoxin were located in an Anchorage hospital and immediately delivered by train to a nearby city, Nenana, en route to Nome. Nenana was the furthest city that was reachable by rail – but unfortunately it was still more than 600 miles outside of Nome, with no transportation to make the delivery. Meanwhile, Welch had confirmed 20 total cases of diphtheria, with dozens more at high risk. Diphtheria was known for wiping out entire communities, and the entire town of Nome was in danger of suffering the same fate.
It was Mark Summer, the Board of Health superintendent, who suggested something unorthodox: Using a relay team of sled-racing dogs to deliver the antitoxin serum from Nenana to Nome. The Board quickly voted to accept Summer’s idea and set up a plan: The thousands of units of antitoxin serum would be passed along from team to team at different towns along the mail route from Nenana to Nome. When it reached a town called Nulato, a famed dogsled racer named Leonhard Seppala and his experienced team of huskies would take the serum more than 90 miles over the ice of Norton Sound, the longest and most treacherous part of the journey. Past the sound, the serum would change hands several times more before arriving in Nome.
Between January 27 and 31, the serum passed through roughly a dozen drivers and their dog sled teams, each of them carrying the serum between 20 and 50 miles to the next destination. Though each leg of the trip took less than a day, the sub-zero temperatures – sometimes as low as -85 degrees – meant that every driver and dog risked their lives. When the first driver, Bill Shannon, arrived at his checkpoint in Tolovana on January 28th, his nose was black with frostbite, and three of his dogs had died. The driver who relieved Bill Shannon, named Edgar Kalland, needed the owner of a local roadhouse to pour hot water over his hands to free them from the sled’s metal handlebar. Two more dogs from another relay team died before the serum was passed to Seppala at a town called Ungalik.
THE FINAL STRETCHES
Seppala and his team raced across the ice of the Norton Sound in the dead of night on January 31, with wind chill temperatures nearing an astonishing -90 degrees. The team traveled 84 miles in a single day before stopping to rest – and once rested, they set off again in the middle of the night through a raging winter storm. The team made it across the ice, as well as a 5,000-foot ascent up Little McKinley Mountain, to pass the serum to another driver in record time. The serum was now just 78 miles from Nome, and the death toll in town had reached 28.
The serum reached Gunnar Kaasen and his team of dogs on February 1st. Balto, Kaasen’s lead dog, guided the team heroically through a winter storm that was so severe Kaasen later reported not being able to see the dogs that were just a few feet ahead of him.
Visibility was so poor, in fact, that Kaasen ran his sled two miles past the relay point before noticing – and not wanting to lose a minute, he decided to forge on ahead rather than doubling back to deliver the serum to another driver. As they continued through the storm, the hurricane-force winds ripped past Kaasen’s sled at one point and toppled the sled – and the serum – overboard. The cylinder containing the antitoxin was left buried in the snow – and Kaasen tore off his gloves and dug through the tundra to locate it. Though it resulted in a bad case of frostbite, Kaasen eventually found the cylinder and kept driving.
Kaasen arrived at the next relay point on February 2nd, hours ahead of schedule. When he got there, however, he found the relay driver of the next team asleep. Kaasen took a risk and decided not to wake him, fearing that time would be wasted with the next driver readying his team. Kaasen, Balto, and the rest of the team forged on, driving another 25 miles before finally reaching Nome just before six in the morning. Eyewitnesses described Kaasen pulling up to the town’s bank and stumbling to the front of the sled. There, he collapsed in exhaustion, telling onlookers that Balto was “a damn fine dog.”
A LIVING LEGACY
Just a few hours after Balto’s heroic arrival in Nome, the serum had been thawed and was ready to administer to the patients with diphtheria. Amazingly, the relay team managed to complete the entire journey in just 127 hours – a world record at the time – without one serum vial damaged or destroyed. The serum shipment that arrived by dogsled – along with additional serum deliveries that followed in the next several weeks – were successful in stopping the outbreak in its tracks.
Balto and several other dogs – including Togo, the lead dog on Seppala’s team – were celebrated as local heroes after the race. Balto died in 1933, while the last of the human serum runners died in 1999 – but their legacy lives on: In early 2021, an all-female team of healthcare workers made the news by braving the Alaskan winter to deliver COVID-19 vaccines to people in rural North Alaska, traveling by bobsled and snowmobile – a heroic journey, and one that would have been unthinkable had Balto, Togo, and the 1925 sled runners not first paved the way.