DNA gathered from animal poop helps protect wildlife
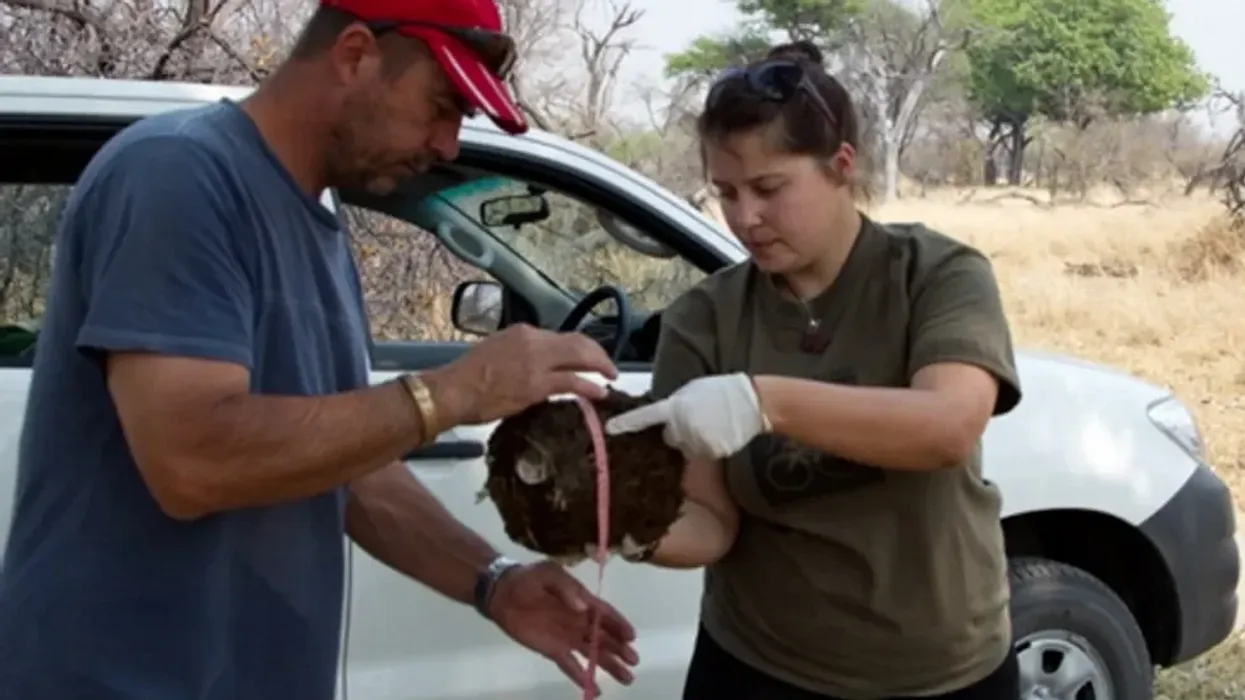
Alida de Flamingh and her team are collecting elephant dung. It holds a trove of information about animal health, diet and genetic diversity.
On the savannah near the Botswana-Zimbabwe border, elephants grazed contentedly. Nearby, postdoctoral researcher Alida de Flamingh watched and waited. As the herd moved away, she went into action, collecting samples of elephant dung that she and other wildlife conservationists would study in the months to come. She pulled on gloves, took a swab, and ran it all over the still-warm, round blob of elephant poop.
Sequencing DNA from fecal matter is a safe, non-invasive way to track and ultimately help protect over 42,000 species currently threatened by extinction. Scientists are using this DNA to gain insights into wildlife health, genetic diversity and even the broader environment. Applied to elephants, chimpanzees, toucans and other species, it helps scientists determine the genetic diversity of groups and linkages with other groups. Such analysis can show changes in rates of inbreeding. Populations with greater genetic diversity adapt better to changes and environmental stressors than those with less diversity, thus reducing their risks of extinction, explains de Flamingh, a postdoctoral researcher at the University of Illinois Urbana-Champaign.
Analyzing fecal DNA also reveals information about an animal’s diet and health, and even nearby flora that is eaten. That information gives scientists broader insights into the ecosystem, and the findings are informing conservation initiatives. Examples include restoring or maintaining genetic connections among groups, ensuring access to certain foraging areas or increasing diversity in captive breeding programs.
Approximately 27 percent of mammals and 28 percent of all assessed species are close to dying out. The IUCN Red List of threatened species, simply called the Red List, is the world’s most comprehensive record of animals’ risk of extinction status. The more information scientists gather, the better their chances of reducing those risks. In Africa, populations of vertebrates declined 69 percent between 1970 and 2022, according to the World Wildlife Fund (WWF).
“We put on sterile gloves and use a sterile swab to collect wet mucus and materials from the outside of the dung ball,” says Alida de Flamingh, a postdoctoral researcher at the University of Illinois Urbana-Champaign.
“When people talk about species, they often talk about ecosystems, but they often overlook genetic diversity,” says Christina Hvilsom, senior geneticist at the Copenhagen Zoo. “It’s easy to count (individuals) to assess whether the population size is increasing or decreasing, but diversity isn’t something we can see with our bare eyes. Yet, it’s actually the foundation for the species and populations.” DNA analysis can provide this critical information.
Assessing elephants’ health
“Africa’s elephant populations are facing unprecedented threats,” says de Flamingh, the postdoc, who has studied them since 2009. Challenges include ivory poaching, habitat destruction and smaller, more fragmented habitats that result in smaller mating pools with less genetic diversity. Additionally, de Flamingh studies the microbial communities living on and in elephants – their microbiomes – looking for parasites or dangerous microbes.
Approximately 415,000 elephants inhabit Africa today, but de Flamingh says the number would be four times higher without these challenges. The IUCN Red List reports African savannah elephants are endangered and African forest elephants are critically endangered. Elephants support ecosystem biodiversity by clearing paths that help other species travel. Their very footprints create small puddles that can host smaller organisms such as tadpoles. Elephants are often described as ecosystems’ engineers, so if they disappear, the rest of the ecosystem will suffer too.
There’s a process to collecting elephant feces. “We put on sterile gloves (which we change for each sample) and use a sterile swab to collect wet mucus and materials from the outside of the dung ball,” says de Flamingh. They rub a sample about the size of a U.S. quarter onto a paper card embedded with DNA preservation technology. Each card is air dried and stored in a packet of desiccant to prevent mold growth. This way, samples can be stored at room temperature indefinitely without the DNA degrading.
Earlier methods required collecting dung in bags, which needed either refrigeration or the addition of preservatives, or the riskier alternative of tranquilizing the animals before approaching them to draw blood samples. The ability to collect and sequence the DNA made things much easier and safer.
“Our research provides a way to assess elephant health without having to physically interact with elephants,” de Flamingh emphasizes. “We also keep track of the GPS coordinates of each sample so that we can create a map of the sampling locations,” she adds. That helps researchers correlate elephants’ health with geographic areas and their conditions.
Although de Flamingh works with elephants in the wild, the contributions of zoos in the United States and collaborations in South Africa (notably the late Professor Rudi van Aarde and the Conservation Ecology Research Unit at the University of Pretoria) were key in studying this method to ensure it worked, she points out.
Protecting chimpanzees
Genetic work with chimpanzees began about a decade ago. Hvilsom and her group at the Copenhagen Zoo analyzed DNA from nearly 1,000 fecal samples collected between 2003 and 2018 by a team of international researchers. The goal was to assess the status of the West African subspecies, which is critically endangered after rapid population declines. Of the four subspecies of chimpanzees, the West African subspecies is considered the most at-risk.
In total, the WWF estimates the numbers of chimpanzees inhabiting Africa’s forests and savannah woodlands at between 173,000 and 300,000. Poaching, disease and human-caused changes to their lands are their major risks.
By analyzing genetics obtained from fecal samples, Hvilsom estimated the chimpanzees’ population, ascertained their family relationships and mapped their migration routes.
“One of the threats is mining near the Nimba Mountains in Guinea,” a stronghold for the West African subspecies, Hvilsom says. The Nimba Mountains are a UNESCO World Heritage Site, but they are rich in iron ore, which is used to make the steel that is vital to the Asian construction boom. As she and colleagues wrote in a recent paper, “Many extractive industries are currently developing projects in chimpanzee habitat.”
Analyzing DNA allows researchers to identify individual chimpanzees more accurately than simply observing them, she says. Normally, field researchers would install cameras and manually inspect each picture to determine how many chimpanzees were in an area. But, Hvilsom says, “That’s very tricky. Chimpanzees move a lot and are fast, so it’s difficult to get clear pictures. Often, they find and destroy the cameras. Also, they live in large areas, so you need a lot of cameras.”
By analyzing genetics obtained from fecal samples, Hvilsom estimated the chimpanzees’ population, ascertained their family relationships and mapped their migration routes based upon DNA comparisons with other chimpanzee groups. The mining companies and builders are using this information to locate future roads where they won’t disrupt migration – a more effective solution than trying to build artificial corridors for wildlife.
“The current route cuts off communities of chimpanzees,” Hvilsom elaborates. That effectively prevents young adult chimps from joining other groups when the time comes, eventually reducing the currently-high levels of genetic diversity.
“The mining company helped pay for the genetics work,” Hvilsom says, “as part of its obligation to assess and monitor biodiversity and the effect of the mining in the area.”
Of 50 toucan subspecies, 11 are threatened or near-threatened with extinction because of deforestation and poaching.
Identifying toucan families
Feces aren't the only substance researchers draw DNA samples from. Jeffrey Coleman, a Ph.D. candidate at the University of Texas at Austin relies on blood tests for studying the genetic diversity of toucans---birds species native to Central America and nearby regions. They live in the jungles, where they hop among branches, snip fruit from trees, toss it in the air and catch it with their large beaks. “Toucans are beautiful, charismatic birds that are really important to the ecosystem,” says Coleman.
Of their 50 subspecies, 11 are threatened or near-threatened with extinction because of deforestation and poaching. “When people see these aesthetically pleasing birds, they’re motivated to care about conservation practices,” he points out.
Coleman works with the Dallas World Aquarium and its partner zoos to analyze DNA from blood draws, using it to identify which toucans are related and how closely. His goal is to use science to improve the genetic diversity among toucan offspring.
Specifically, he’s looking at sections of the genome of captive birds in which the nucleotides repeat multiple times, such as AGATAGATAGAT. Called microsatellites, these consecutively-repeating sections can be passed from parents to children, helping scientists identify parent-child and sibling-sibling relationships. “That allows you to make strategic decisions about how to pair (captive) individuals for mating...to avoid inbreeding,” Coleman says.
Jeffrey Coleman is studying the microsatellites inside the toucan genomes.
Courtesy Jeffrey Coleman
The alternative is to use a type of analysis that looks for a single DNA building block – a nucleotide – that differs in a given sequence. Called single nucleotide polymorphisms (SNPs, pronounced “snips”), they are very common and very accurate. Coleman says they are better than microsatellites for some uses. But scientists have already developed a large body of microsatellite data from multiple species, so microsatellites can shed more insights on relations.
Regardless of whether conservation programs use SNPs or microsatellites to guide captive breeding efforts, the goal is to help them build genetically diverse populations that eventually may supplement endangered populations in the wild. “The hope is that the ecosystem will be stable enough and that the populations (once reintroduced into the wild) will be able to survive and thrive,” says Coleman. History knows some good examples of captive breeding success.
The California condor, which had a total population of 27 in 1987, when the last wild birds were captured, is one of them. A captive breeding program boosted their numbers to 561 by the end of 2022. Of those, 347 of those are in the wild, according to the National Park Service.
Conservationists hope that their work on animals’ genetic diversity will help preserve and restore endangered species in captivity and the wild. DNA analysis is crucial to both types of efforts. The ability to apply genome sequencing to wildlife conservation brings a new level of accuracy that helps protect species and gives fresh insights that observation alone can’t provide.
“A lot of species are threatened,” Coleman says. “I hope this research will be a resource people can use to get more information on longer-term genealogies and different populations.”
How the Human Brain Project Built a Mind of its Own
In 2013, the Human Brain Project set out to build a realistic computer model of the brain over ten years. Now, experts are reflecting on HBP's achievements with an eye toward the future.
In 2009, neuroscientist Henry Markram gave an ambitious TED talk. “Our mission is to build a detailed, realistic computer model of the human brain,” he said, naming three reasons for this unmatched feat of engineering. One was because understanding the human brain was essential to get along in society. Another was because experimenting on animal brains could only get scientists so far in understanding the human ones. Third, medicines for mental disorders weren’t good enough. “There are two billion people on the planet that are affected by mental disorders, and the drugs that are used today are largely empirical,” Markram said. “I think that we can come up with very concrete solutions on how to treat disorders.”
Markram's arguments were very persuasive. In 2013, the European Commission launched the Human Brain Project, or HBP, as part of its Future and Emerging Technologies program. Viewed as Europe’s chance to try to win the “brain race” between the U.S., China, Japan, and other countries, the project received about a billion euros in funding with the goal to simulate the entire human brain on a supercomputer, or in silico, by 2023.
Now, after 10 years of dedicated neuroscience research, the HBP is coming to an end. As its many critics warned, it did not manage to build an entire human brain in silico. Instead, it achieved a multifaceted array of different goals, some of them unexpected.
Scholars have found that the project did help advance neuroscience more than some detractors initially expected, specifically in the area of brain simulations and virtual models. Using an interdisciplinary approach of combining technology, such as AI and digital simulations, with neuroscience, the HBP worked to gain a deeper understanding of the human brain’s complicated structure and functions, which in some cases led to novel treatments for brain disorders. Lastly, through online platforms, the HBP spearheaded a previously unmatched level of global neuroscience collaborations.
Simulating a human brain stirs up controversy
Right from the start, the project was plagued with controversy and condemnation. One of its prominent critics was Yves Fregnac, a professor in cognitive science at the Polytechnic Institute of Paris and research director at the French National Centre for Scientific Research. Fregnac argued in numerous articles that the HBP was overfunded based on proposals with unrealistic goals. “This new way of over-selling scientific targets, deeply aligned with what modern society expects from mega-sciences in the broad sense (big investment, big return), has been observed on several occasions in different scientific sub-fields,” he wrote in one of his articles, “before invading the field of brain sciences and neuromarketing.”
"A human brain model can simulate an experiment a million times for many different conditions, but the actual human experiment can be performed only once or a few times," said Viktor Jirsa, a professor at Aix-Marseille University.
Responding to such critiques, the HBP worked to restructure the effort in its early days with new leadership, organization, and goals that were more flexible and attainable. “The HBP got a more versatile, pluralistic approach,” said Viktor Jirsa, a professor at Aix-Marseille University and one of the HBP lead scientists. He believes that these changes fixed at least some of HBP’s issues. “The project has been on a very productive and scientifically fruitful course since then.”
After restructuring, the HBP became a European hub on brain research, with hundreds of scientists joining its growing network. The HBP created projects focused on various brain topics, from consciousness to neurodegenerative diseases. HBP scientists worked on complex subjects, such as mapping out the brain, combining neuroscience and robotics, and experimenting with neuromorphic computing, a computational technique inspired by the human brain structure and function—to name just a few.
Simulations advance knowledge and treatment options
In 2013, it seemed that bringing neuroscience into a digital age would be farfetched, but research within the HBP has made this achievable. The virtual maps and simulations various HBP teams create through brain imaging data make it easier for neuroscientists to understand brain developments and functions. The teams publish these models on the HBP’s EBRAINS online platform—one of the first to offer access to such data to neuroscientists worldwide via an open-source online site. “This digital infrastructure is backed by high-performance computers, with large datasets and various computational tools,” said Lucy Xiaolu Wang, an assistant professor in the Resource Economics Department at the University of Massachusetts Amherst, who studies the economics of the HBP. That means it can be used in place of many different types of human experimentation.
Jirsa’s team is one of many within the project that works on virtual brain models and brain simulations. Compiling patient data, Jirsa and his team can create digital simulations of different brain activities—and repeat these experiments many times, which isn’t often possible in surgeries on real brains. “A human brain model can simulate an experiment a million times for many different conditions,” Jirsa explained, “but the actual human experiment can be performed only once or a few times.” Using simulations also saves scientists and doctors time and money when looking at ways to diagnose and treat patients with brain disorders.
Compiling patient data, scientists can create digital simulations of different brain activities—and repeat these experiments many times.
The Human Brain Project
Simulations can help scientists get a full picture that otherwise is unattainable. “Another benefit is data completion,” added Jirsa, “in which incomplete data can be complemented by the model. In clinical settings, we can often measure only certain brain areas, but when linked to the brain model, we can enlarge the range of accessible brain regions and make better diagnostic predictions.”
With time, Jirsa’s team was able to move into patient-specific simulations. “We advanced from generic brain models to the ability to use a specific patient’s brain data, from measurements like MRI and others, to create individualized predictive models and simulations,” Jirsa explained. He and his team are working on this personalization technique to treat patients with epilepsy. According to the World Health Organization, about 50 million people worldwide suffer from epilepsy, a disorder that causes recurring seizures. While some epilepsy causes are known others remain an enigma, and many are hard to treat. For some patients whose epilepsy doesn’t respond to medications, removing part of the brain where seizures occur may be the only option. Understanding where in the patients’ brains seizures arise can give scientists a better idea of how to treat them and whether to use surgery versus medications.
“We apply such personalized models…to precisely identify where in a patient’s brain seizures emerge,” Jirsa explained. “This guides individual surgery decisions for patients for which surgery is the only treatment option.” He credits the HBP for the opportunity to develop this novel approach. “The personalization of our epilepsy models was only made possible by the Human Brain Project, in which all the necessary tools have been developed. Without the HBP, the technology would not be in clinical trials today.”
Personalized simulations can significantly advance treatments, predict the outcome of specific medical procedures and optimize them before actually treating patients. Jirsa is watching this happen firsthand in his ongoing research. “Our technology for creating personalized brain models is now used in a large clinical trial for epilepsy, funded by the French state, where we collaborate with clinicians in hospitals,” he explained. “We have also founded a spinoff company called VB Tech (Virtual Brain Technologies) to commercialize our personalized brain model technology and make it available to all patients.”
The Human Brain Project created a level of interconnectedness within the neuroscience research community that never existed before—a network not unlike the brain’s own.
Other experts believe it’s too soon to tell whether brain simulations could change epilepsy treatments. “The life cycle of developing treatments applicable to patients often runs over a decade,” Wang stated. “It is still too early to draw a clear link between HBP’s various project areas with patient care.” However, she admits that some studies built on the HBP-collected knowledge are already showing promise. “Researchers have used neuroscientific atlases and computational tools to develop activity-specific stimulation programs that enabled paraplegic patients to move again in a small-size clinical trial,” Wang said. Another intriguing study looked at simulations of Alzheimer’s in the brain to understand how it evolves over time.
Some challenges remain hard to overcome even with computer simulations. “The major challenge has always been the parameter explosion, which means that many different model parameters can lead to the same result,” Jirsa explained. An example of this parameter explosion could be two different types of neurodegenerative conditions, such as Parkinson’s and Huntington’s diseases. Both afflict the same area of the brain, the basal ganglia, which can affect movement, but are caused by two different underlying mechanisms. “We face the same situation in the living brain, in which a large range of diverse mechanisms can produce the same behavior,” Jirsa said. The simulations still have to overcome the same challenge.
Understanding where in the patients’ brains seizures arise can give scientists a better idea of how to treat them and whether to use surgery versus medications.
The Human Brain Project
A network not unlike the brain’s own
Though the HBP will be closing this year, its legacy continues in various studies, spin-off companies, and its online platform, EBRAINS. “The HBP is one of the earliest brain initiatives in the world, and the 10-year long-term goal has united many researchers to collaborate on brain sciences with advanced computational tools,” Wang said. “Beyond the many research articles and projects collaborated on during the HBP, the online neuroscience research infrastructure EBRAINS will be left as a legacy even after the project ends.”
Those who worked within the HBP see the end of this project as the next step in neuroscience research. “Neuroscience has come closer to very meaningful applications through the systematic link with new digital technologies and collaborative work,” Jirsa stated. “In that way, the project really had a pioneering role.” It also created a level of interconnectedness within the neuroscience research community that never existed before—a network not unlike the brain’s own. “Interconnectedness is an important advance and prerequisite for progress,” Jirsa said. “The neuroscience community has in the past been rather fragmented and this has dramatically changed in recent years thanks to the Human Brain Project.”
According to its website, by 2023 HBP’s network counted over 500 scientists from over 123 institutions and 16 different countries, creating one of the largest multi-national research groups in the world. Even though the project hasn’t produced the in-silico brain as Markram envisioned it, the HBP created a communal mind with immense potential. “It has challenged us to think beyond the boundaries of our own laboratories,” Jirsa said, “and enabled us to go much further together than we could have ever conceived going by ourselves.”
Regenerative medicine has come a long way, baby
After a cloned baby sheep, what started as one of the most controversial areas in medicine is now promising to transform it.
The field of regenerative medicine had a shaky start. In 2002, when news spread about the first cloned animal, Dolly the sheep, a raucous debate ensued. Scary headlines and organized opposition groups put pressure on government leaders, who responded by tightening restrictions on this type of research.
Fast forward to today, and regenerative medicine, which focuses on making unhealthy tissues and organs healthy again, is rewriting the code to healing many disorders, though it’s still young enough to be considered nascent. What started as one of the most controversial areas in medicine is now promising to transform it.
Progress in the lab has addressed previous concerns. Back in the early 2000s, some of the most fervent controversy centered around somatic cell nuclear transfer (SCNT), the process used by scientists to produce Dolly. There was fear that this technique could be used in humans, with possibly adverse effects, considering the many medical problems of the animals who had been cloned.
But today, scientists have discovered better approaches with fewer risks. Pioneers in the field are embracing new possibilities for cellular reprogramming, 3D organ printing, AI collaboration, and even growing organs in space. It could bring a new era of personalized medicine for longer, healthier lives - while potentially sparking new controversies.
Engineering tissues from amniotic fluids
Work in regenerative medicine seeks to reverse damage to organs and tissues by culling, modifying and replacing cells in the human body. Scientists in this field reach deep into the mechanisms of diseases and the breakdowns of cells, the little workhorses that perform all life-giving processes. If cells can’t do their jobs, they take whole organs and systems down with them. Regenerative medicine seeks to harness the power of healthy cells derived from stem cells to do the work that can literally restore patients to a state of health—by giving them healthy, functioning tissues and organs.
Modern-day regenerative medicine takes its origin from the 1998 isolation of human embryonic stem cells, first achieved by John Gearhart at Johns Hopkins University. Gearhart isolated the pluripotent cells that can differentiate into virtually every kind of cell in the human body. There was a raging controversy about the use of these cells in research because at that time they came exclusively from early-stage embryos or fetal tissue.
Back then, the highly controversial SCNT cells were the only way to produce genetically matched stem cells to treat patients. Since then, the picture has changed radically because other sources of highly versatile stem cells have been developed. Today, scientists can derive stem cells from amniotic fluid or reprogram patients’ skin cells back to an immature state, so they can differentiate into whatever types of cells the patient needs.
In the context of medical history, the field of regenerative medicine is progressing at a dizzying speed. But for those living with aggressive or chronic illnesses, it can seem that the wheels of medical progress grind slowly.
The ethical debate has been dialed back and, in the last few decades, the field has produced important innovations, spurring the development of whole new FDA processes and categories, says Anthony Atala, a bioengineer and director of the Wake Forest Institute for Regenerative Medicine. Atala and a large team of researchers have pioneered many of the first applications of 3D printed tissues and organs using cells developed from patients or those obtained from amniotic fluid or placentas.
His lab, considered to be the largest devoted to translational regenerative medicine, is currently working with 40 different engineered human tissues. Sixteen of them have been transplanted into patients. That includes skin, bladders, urethras, muscles, kidneys and vaginal organs, to name just a few.
These achievements are made possible by converging disciplines and technologies, such as cell therapies, bioengineering, gene editing, nanotechnology and 3D printing, to create living tissues and organs for human transplants. Atala is currently overseeing clinical trials to test the safety of tissues and organs engineered in the Wake Forest lab, a significant step toward FDA approval.
In the context of medical history, the field of regenerative medicine is progressing at a dizzying speed. But for those living with aggressive or chronic illnesses, it can seem that the wheels of medical progress grind slowly.
“It’s never fast enough,” Atala says. “We want to get new treatments into the clinic faster, but the reality is that you have to dot all your i’s and cross all your t’s—and rightly so, for the sake of patient safety. People want predictions, but you can never predict how much work it will take to go from conceptualization to utilization.”
As a surgeon, he also treats patients and is able to follow transplant recipients. “At the end of the day, the goal is to get these technologies into patients, and working with the patients is a very rewarding experience,” he says. Will the 3D printed organs ever outrun the shortage of donated organs? “That’s the hope,” Atala says, “but this technology won’t eliminate the need for them in our lifetime.”
New methods are out of this world
Jeanne Loring, another pioneer in the field and director of the Center for Regenerative Medicine at Scripps Research Institute in San Diego, says that investment in regenerative medicine is not only paying off, but is leading to truly personalized medicine, one of the holy grails of modern science.
This is because a patient’s own skin cells can be reprogrammed to become replacements for various malfunctioning cells causing incurable diseases, such as diabetes, heart disease, macular degeneration and Parkinson’s. If the cells are obtained from a source other than the patient, they can be rejected by the immune system. This means that patients need lifelong immunosuppression, which isn’t ideal. “With Covid,” says Loring, “I became acutely aware of the dangers of immunosuppression.” Using the patient’s own cells eliminates that problem.
Microgravity conditions make it easier for the cells to form three-dimensional structures, which could more easily lead to the growing of whole organs. In fact, Loring's own cells have been sent to the ISS for study.
Loring has a special interest in neurons, or brain cells that can be developed by manipulating cells found in the skin. She is looking to eventually treat Parkinson’s disease using them. The manipulated cells produce dopamine, the critical hormone or neurotransmitter lacking in the brains of patients. A company she founded plans to start a Phase I clinical trial using cell therapies for Parkinson’s soon, she says.
This is the culmination of many years of basic research on her part, some of it on her own cells. In 2007, Loring had her own cells reprogrammed, so there’s a cell line that carries her DNA. “They’re just like embryonic stem cells, but personal,” she said.
Loring has another special interest—sending immature cells into space to be studied at the International Space Station. There, microgravity conditions make it easier for the cells to form three-dimensional structures, which could more easily lead to the growing of whole organs. In fact, her own cells have been sent to the ISS for study. “My colleagues and I have completed four missions at the space station,” she says. “The last cells came down last August. They were my own cells reprogrammed into pluripotent cells in 2009. No one else can say that,” she adds.
Future controversies and tipping points
Although the original SCNT debate has calmed down, more controversies may arise, Loring thinks.
One of them could concern growing synthetic embryos. The embryos are ultimately derived from embryonic stem cells, and it’s not clear to what stage these embryos can or will be grown in an artificial uterus—another recent invention. The science, so far done only in animals, is still new and has not been widely publicized but, eventually, “People will notice the production of synthetic embryos and growing them in an artificial uterus,” Loring says. It’s likely to incite many of the same reactions as the use of embryonic stem cells.
Bernard Siegel, the founder and director of the Regenerative Medicine Foundation and executive director of the newly formed Healthspan Action Coalition (HSAC), believes that stem cell science is rapidly approaching tipping point and changing all of medical science. (For disclosure, I do consulting work for HSAC). Siegel says that regenerative medicine has become a new pillar of medicine that has recently been fast-tracked by new technology.
Artificial intelligence is speeding up discoveries and the convergence of key disciplines, as demonstrated in Atala’s lab, which is creating complex new medical products that replace the body’s natural parts. Just as importantly, those parts are genetically matched and pose no risk of rejection.
These new technologies must be regulated, which can be a challenge, Siegel notes. “Cell therapies represent a challenge to the existing regulatory structure, including payment, reimbursement and infrastructure issues that 20 years ago, didn’t exist.” Now the FDA and other agencies are faced with this revolution, and they’re just beginning to adapt.
Siegel cited the 2021 FDA Modernization Act as a major step. The Act allows drug developers to use alternatives to animal testing in investigating the safety and efficacy of new compounds, loosening the agency’s requirement for extensive animal testing before a new drug can move into clinical trials. The Act is a recognition of the profound effect that cultured human cells are having on research. Being able to test drugs using actual human cells promises to be far safer and more accurate in predicting how they will act in the human body, and could accelerate drug development.
Siegel, a longtime veteran and founding father of several health advocacy organizations, believes this work helped bring cell therapies to people sooner rather than later. His new focus, through the HSAC, is to leverage regenerative medicine into extending not just the lifespan but the worldwide human healthspan, the period of life lived with health and vigor. “When you look at the HSAC as a tree,” asks Siegel, “what are the roots of that tree? Stem cell science and the huge ecosystem it has created.” The study of human aging is another root to the tree that has potential to lengthen healthspans.
The revolutionary science underlying the extension of the healthspan needs to be available to the whole world, Siegel says. “We need to take all these roots and come up with a way to improve the life of all mankind,” he says. “Everyone should be able to take advantage of this promising new world.”