Hacking Your Own Genes: A Recipe for Disaster
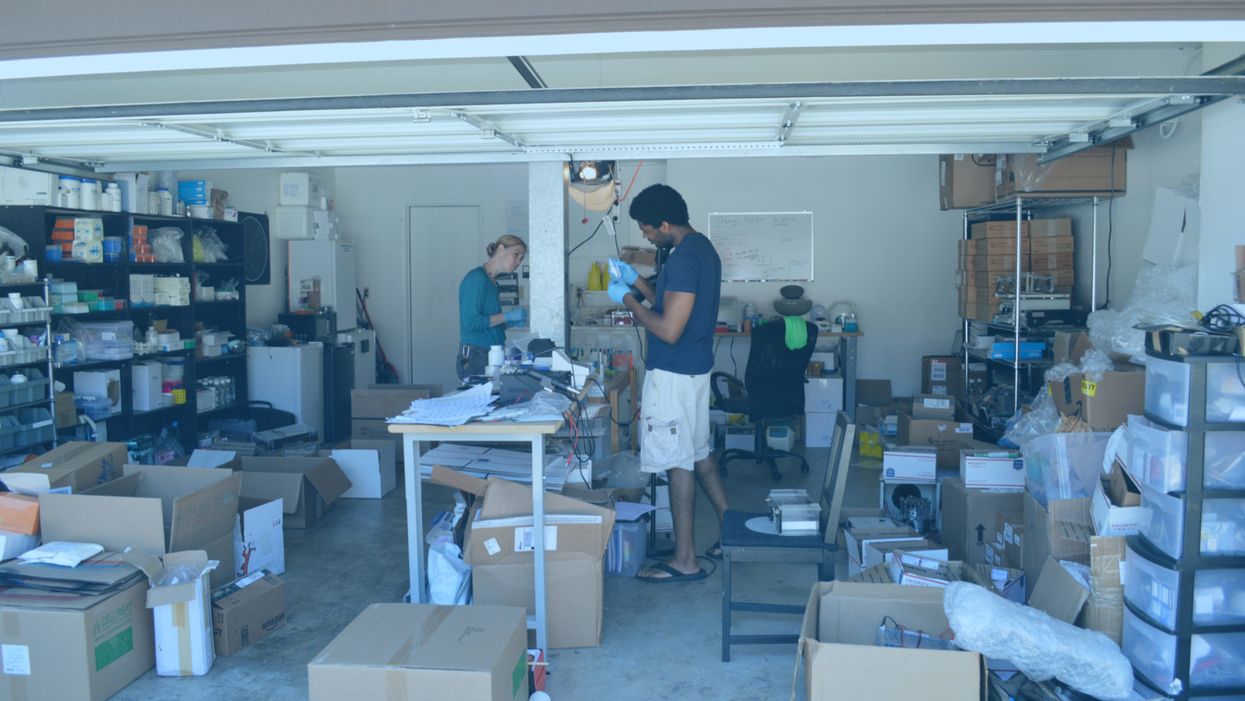
Employees of ODIN, a consumer genetic design and engineering company, working out of their Bay Area garage start-up lab in 2016.
Editor's Note: Our Big Moral Question this month is: "Where should we draw a line, if any, between the use of gene editing for the prevention and treatment of disease, and for cosmetic enhancement?" It is illegal in the U.S. to develop human trials for the latter, even though some people think it should be acceptable. The most outspoken supporter recently resorted to self-experimentation using CRISPR in his own makeshift lab. But critics argue that "biohackers" like him are recklessly courting harm. LeapsMag invited a leading intellectual from the Center for Genetics and Society to share her perspective.
"I want to democratize science," says biohacker extraordinaire Josiah Zayner.
This is certainly a worthy-sounding sentiment. And it is central to the ethos of biohacking, a term that's developed a bit of sprawl. Biohacking can mean non-profit community biology labs that promote "citizen science," or clever but not necessarily safe or innocuous garage-based experiments with computers and genetics, or efforts at biological self-optimization via techniques including cybernetic implants, drug supplements, and intermittent fasting.
They appear to have given little thought to whether curiosity should be bound in any way by care for social consequence.
Against that messy background, what should we make of Zayner? The thirty-something ex-NASA scientist, who describes himself as "a global leader in the BioHacker movement," put his interpretation of democracy on display last October during a CRISPR-yourself performance at a San Francisco biotech conference. In that episode, he dramatically jabbed himself with a long needle, injecting his left forearm with a home-made gene-editing concoction that he said would disrupt his myostatin genes and bulk up his muscles.
Zayner sees himself, and is seen by some fellow biohackers, as a rebel hero: an intrepid scientific adventurer willing to risk his own well-being in the tradition of self-experimentation, eager to push the boundaries of established science in the service of forging innovative modes of discovery, ready to stand up to those stodgy bureaucrats at the FDA in the name of biohacker freedom.
To others, including some in the biohacker community, he's a publicity-seeking stunt man, perhaps deluded by touches of toxic masculinity and techno-entrepreneurial ideology, peddling snake-oil with oozing ramifications.
Zayner is hardly coy about his goals being larger than Popeye-like muscles. "I want to live in a world where people are genetically modifying themselves," he told FastCompany. "I think this is, like, literally, a new era of human beings," he mused to CBS in November. "It's gonna create a whole new species of humans."
Nor does he deign to conceal his tactics. The webpage of the company he launched to sell DIY gene-editing kits (which is advised by celebrity geneticist George Church) says that Zayner is "constantly pushing the boundaries of Science outside traditional environments." He is more explicit when performing: "Yes I am a criminal. And my crime is that of curiosity," he said last August to a biohacker audience in Oakland, which according to Gizmodo erupted in applause.
Regrettably, Zayner, along with some other biohackers and their defenders in the mainstream scientific world, appear to have given little thought to whether curiosity should be bound in any way by care for social consequence.
In December, the FDA issued a brief statement warning against using DIY kits for self-administered gene editing.
Though what's most directly at risk in Zayner's self-enhancement hack is his own safety, his bad-boy celebrity status is likely to encourage emulation. A few weeks after his San Francisco performance, 27-year-old Tristan Roberts took to Facebook Live to give himself a DIY gene modification injection to keep his HIV infection in check, because he doesn't like taking the regular medications that prevent AIDS. Whatever it was that he put into his body was provided by a company that Gizmodo describes as a "mysterious biotech firm with transhumanist leanings."
Zayner doesn't outright provide DIY gene hacks to others. But among his company's offerings are a free DIY Human CRISPR Guide and a $20 CRISPR-Cas9 plasmid that targets the human myostatin gene – the one that Zayner said he was targeting to make his muscles grow. Presumably to fend off legal problems, the product page says: "This product is not injectable or meant for direct human use" – a label as toothless as the fine print on cigarette packages that breaks the news that smoking causes cancer.
Some scientists warn that Zayner's style of biohacking carries considerable dangers. Microbiologist Brian Hanley, himself a self-experimenter who now opposes "biohacking humans," focuses on the technical difficulty of purifying what's being injected. "Screwing up can kill you from endotoxin," he says. "If you get in trouble, call me. I will do my best to instruct the physician how to save your life….But I make no guarantees you will survive."
Hanley also commented on the likely effectiveness of Zayner's effort: "Either Josiah Zayner is ignorant or he is deliberately misleading people. What he suggests cannot work as advertised."
Ensuring the safety and effectiveness of medical drugs and devices is the mandate of the US Food and Drug Administration. In December, the agency issued a brief statement warning against using DIY kits for self-administered gene editing, and saying flat out that selling them is against the law.
The stem cell field provides an unfortunate model of what can go wrong.
Zayner is dismissive of the safety risks. He asks in a Buzzfeed article whether DIY CRISPR should be considered more harmful than smoking or chemotherapy, "legal and socially acceptable activities that damage your genes." This is a strange line of argument, given the decades-long battles with the tobacco industry to raise awareness about smoking's significant harms, and since the side effects of chemotherapy are typically not undertaken by choice.
But the implications of what Zayner, Roberts, and some of their fellow biohackers are promoting ripple well beyond direct harms to individuals. Their rhetoric and vision affect the larger project of biomedicine, and the fraught relationships among drug researchers, pharmaceutical companies, clinical trial subjects, patients, and the public. Writing in Scientific American, Eleanor Pauwels of the Wilson Center, who is sympathetic to biohacking, lists the down sides: "blurred boundaries between treatments and self-experimentation, peer pressure to participate in trials, exploitation of vulnerable individuals, lack of oversight concerning quality control and risk of harm, and more."
These prospects are germane to the current state of human gene editing. After decades of dashed hopes, including deaths of research subjects, "gene therapy" may now be close to deserving the promise in its name. But with safety and efficacy still being evaluated, it's especially crucial to be honest about limitations as well as possibilities.
The stem cell field provides an unfortunate model of what can go wrong. Fifteen years ago, scientists, patient advocates, and even politicians routinely indulged in wildly over-optimistic enthusiasm about the imminence of stem cell therapies. That binge of irresponsible promotion helped create the current situation of widespread stem cell fraud: hundreds of clinics in the US alone selling unproven treatments to unsuspecting and sometimes desperate patients. Many have had their wallets lightened; some have gone blind or developed strange tumors that doctors have never before seen. The FDA is scrambling to address this still-worsening situation.
Zayner-style biohacking and promotion may also impact the ongoing controversy about whether new gene editing tools should be used in human reproduction to pre-determine the traits of future children and generations. Much of the widespread opposition to "human germline modification" is grounded in concern that it would lead to a society in which real or purported genetic advantages, marketed by fertility clinics to affluent parents, would exacerbate our already shameful levels of inequality and discrimination.
With powerful new technologies increasingly shaping the world, there's a lot riding on our capacity to democratize science. But as a society we don't yet have much practice at it.
Yet Zayner is all for it. In an interview in The Guardian, he comments, "DNA defines what a species is, and I imagine it wouldn't be too long into the future when the human species almost becomes a new species because of these modifications." He notes in a blog post, "We want to grow as a species and maybe change as a species. Whether that is curing disease or immortality or mutant powers is up to you."
This brings us back to Zayner's claim that he is working to democratize science.
The conviction that gene editing involves social and political challenges, not just technical matters, has been voiced at all points on the spectrum of perspective and uncertainty. But Zayner says there's been enough talk. "I want people to stop arguing about whether it's okay to use CRISPR or not use CRISPR….It's too late: I already made the choice for you. Argument over. Let's get on with it now. Let's use this to help people. Or to give people purple skin." (Emphasis added, in case there's any doubt about Zayner's commitment to democracy.)
With powerful new technologies increasingly shaping the world, there's a lot riding on our capacity to democratize science. But as a society we don't yet have much practice at it. In fact, we're not very sure what it would look like. It would clearly mean, as Arizona State University political scientist David Guston puts it, "considering the societal outcomes of research at least as attentively as the scientific and technological outputs." It would need broad participation and demand hard work.
The involvement of serious citizen scientists in such efforts, biohackers included, could be a very good thing. But Zayner's contributions to date have not been helpful.
[Ed. Note: Check out Zayner's perspective: "Genetic Engineering for All: The Last Great Frontier of Human Freedom." Then follow LeapsMag on social media to share your opinion.]
DNA- and RNA-based electronic implants may revolutionize healthcare
The test tubes contain tiny DNA/enzyme-based circuits, which comprise TRUMPET, a new type of electronic device, smaller than a cell.
Implantable electronic devices can significantly improve patients’ quality of life. A pacemaker can encourage the heart to beat more regularly. A neural implant, usually placed at the back of the skull, can help brain function and encourage higher neural activity. Current research on neural implants finds them helpful to patients with Parkinson’s disease, vision loss, hearing loss, and other nerve damage problems. Several of these implants, such as Elon Musk’s Neuralink, have already been approved by the FDA for human use.
Yet, pacemakers, neural implants, and other such electronic devices are not without problems. They require constant electricity, limited through batteries that need replacements. They also cause scarring. “The problem with doing this with electronics is that scar tissue forms,” explains Kate Adamala, an assistant professor of cell biology at the University of Minnesota Twin Cities. “Anytime you have something hard interacting with something soft [like muscle, skin, or tissue], the soft thing will scar. That's why there are no long-term neural implants right now.” To overcome these challenges, scientists are turning to biocomputing processes that use organic materials like DNA and RNA. Other promised benefits include “diagnostics and possibly therapeutic action, operating as nanorobots in living organisms,” writes Evgeny Katz, a professor of bioelectronics at Clarkson University, in his book DNA- And RNA-Based Computing Systems.
While a computer gives these inputs in binary code or "bits," such as a 0 or 1, biocomputing uses DNA strands as inputs, whether double or single-stranded, and often uses fluorescent RNA as an output.
Adamala’s research focuses on developing such biocomputing systems using DNA, RNA, proteins, and lipids. Using these molecules in the biocomputing systems allows the latter to be biocompatible with the human body, resulting in a natural healing process. In a recent Nature Communications study, Adamala and her team created a new biocomputing platform called TRUMPET (Transcriptional RNA Universal Multi-Purpose GatE PlaTform) which acts like a DNA-powered computer chip. “These biological systems can heal if you design them correctly,” adds Adamala. “So you can imagine a computer that will eventually heal itself.”
The basics of biocomputing
Biocomputing and regular computing have many similarities. Like regular computing, biocomputing works by running information through a series of gates, usually logic gates. A logic gate works as a fork in the road for an electronic circuit. The input will travel one way or another, giving two different outputs. An example logic gate is the AND gate, which has two inputs (A and B) and two different results. If both A and B are 1, the AND gate output will be 1. If only A is 1 and B is 0, the output will be 0 and vice versa. If both A and B are 0, the result will be 0. While a computer gives these inputs in binary code or "bits," such as a 0 or 1, biocomputing uses DNA strands as inputs, whether double or single-stranded, and often uses fluorescent RNA as an output. In this case, the DNA enters the logic gate as a single or double strand.
If the DNA is double-stranded, the system “digests” the DNA or destroys it, which results in non-fluorescence or “0” output. Conversely, if the DNA is single-stranded, it won’t be digested and instead will be copied by several enzymes in the biocomputing system, resulting in fluorescent RNA or a “1” output. And the output for this type of binary system can be expanded beyond fluorescence or not. For example, a “1” output might be the production of the enzyme insulin, while a “0” may be that no insulin is produced. “This kind of synergy between biology and computation is the essence of biocomputing,” says Stephanie Forrest, a professor and the director of the Biodesign Center for Biocomputing, Security and Society at Arizona State University.
Biocomputing circles are made of DNA, RNA, proteins and even bacteria.
Evgeny Katz
The TRUMPET’s promise
Depending on whether the biocomputing system is placed directly inside a cell within the human body, or run in a test-tube, different environmental factors play a role. When an output is produced inside a cell, the cell's natural processes can amplify this output (for example, a specific protein or DNA strand), creating a solid signal. However, these cells can also be very leaky. “You want the cells to do the thing you ask them to do before they finish whatever their businesses, which is to grow, replicate, metabolize,” Adamala explains. “However, often the gate may be triggered without the right inputs, creating a false positive signal. So that's why natural logic gates are often leaky." While biocomputing outside a cell in a test tube can allow for tighter control over the logic gates, the outputs or signals cannot be amplified by a cell and are less potent.
TRUMPET, which is smaller than a cell, taps into both cellular and non-cellular biocomputing benefits. “At its core, it is a nonliving logic gate system,” Adamala states, “It's a DNA-based logic gate system. But because we use enzymes, and the readout is enzymatic [where an enzyme replicates the fluorescent RNA], we end up with signal amplification." This readout means that the output from the TRUMPET system, a fluorescent RNA strand, can be replicated by nearby enzymes in the platform, making the light signal stronger. "So it combines the best of both worlds,” Adamala adds.
These organic-based systems could detect cancer cells or low insulin levels inside a patient’s body.
The TRUMPET biocomputing process is relatively straightforward. “If the DNA [input] shows up as single-stranded, it will not be digested [by the logic gate], and you get this nice fluorescent output as the RNA is made from the single-stranded DNA, and that's a 1,” Adamala explains. "And if the DNA input is double-stranded, it gets digested by the enzymes in the logic gate, and there is no RNA created from the DNA, so there is no fluorescence, and the output is 0." On the story's leading image above, if the tube is "lit" with a purple color, that is a binary 1 signal for computing. If it's "off" it is a 0.
While still in research, TRUMPET and other biocomputing systems promise significant benefits to personalized healthcare and medicine. These organic-based systems could detect cancer cells or low insulin levels inside a patient’s body. The study’s lead author and graduate student Judee Sharon is already beginning to research TRUMPET's ability for earlier cancer diagnoses. Because the inputs for TRUMPET are single or double-stranded DNA, any mutated or cancerous DNA could theoretically be detected from the platform through the biocomputing process. Theoretically, devices like TRUMPET could be used to detect cancer and other diseases earlier.
Adamala sees TRUMPET not only as a detection system but also as a potential cancer drug delivery system. “Ideally, you would like the drug only to turn on when it senses the presence of a cancer cell. And that's how we use the logic gates, which work in response to inputs like cancerous DNA. Then the output can be the production of a small molecule or the release of a small molecule that can then go and kill what needs killing, in this case, a cancer cell. So we would like to develop applications that use this technology to control the logic gate response of a drug’s delivery to a cell.”
Although platforms like TRUMPET are making progress, a lot more work must be done before they can be used commercially. “The process of translating mechanisms and architecture from biology to computing and vice versa is still an art rather than a science,” says Forrest. “It requires deep computer science and biology knowledge,” she adds. “Some people have compared interdisciplinary science to fusion restaurants—not all combinations are successful, but when they are, the results are remarkable.”
Crickets are low on fat, high on protein, and can be farmed sustainably. They are also crunchy.
In today’s podcast episode, Leaps.org Deputy Editor Lina Zeldovich speaks about the health and ecological benefits of farming crickets for human consumption with Bicky Nguyen, who joins Lina from Vietnam. Bicky and her business partner Nam Dang operate an insect farm named CricketOne. Motivated by the idea of sustainable and healthy protein production, they started their unconventional endeavor a few years ago, despite numerous naysayers who didn’t believe that humans would ever consider munching on bugs.
Yet, making creepy crawlers part of our diet offers many health and planetary advantages. Food production needs to match the rise in global population, estimated to reach 10 billion by 2050. One challenge is that some of our current practices are inefficient, polluting and wasteful. According to nonprofit EarthSave.org, it takes 2,500 gallons of water, 12 pounds of grain, 35 pounds of topsoil and the energy equivalent of one gallon of gasoline to produce one pound of feedlot beef, although exact statistics vary between sources.
Meanwhile, insects are easy to grow, high on protein and low on fat. When roasted with salt, they make crunchy snacks. When chopped up, they transform into delicious pâtes, says Bicky, who invents her own cricket recipes and serves them at industry and public events. Maybe that’s why some research predicts that edible insects market may grow to almost $10 billion by 2030. Tune in for a delectable chat on this alternative and sustainable protein.
Listen on Apple | Listen on Spotify | Listen on Stitcher | Listen on Amazon | Listen on Google
Further reading:
More info on Bicky Nguyen
https://yseali.fulbright.edu.vn/en/faculty/bicky-n...
The environmental footprint of beef production
https://www.earthsave.org/environment.htm
https://www.watercalculator.org/news/articles/beef-king-big-water-footprints/
https://www.frontiersin.org/articles/10.3389/fsufs.2019.00005/full
https://ourworldindata.org/carbon-footprint-food-methane
Insect farming as a source of sustainable protein
https://www.insectgourmet.com/insect-farming-growing-bugs-for-protein/
https://www.sciencedirect.com/topics/agricultural-and-biological-sciences/insect-farming
Cricket flour is taking the world by storm
https://www.cricketflours.com/
https://talk-commerce.com/blog/what-brands-use-cricket-flour-and-why/
Lina Zeldovich has written about science, medicine and technology for Popular Science, Smithsonian, National Geographic, Scientific American, Reader’s Digest, the New York Times and other major national and international publications. A Columbia J-School alumna, she has won several awards for her stories, including the ASJA Crisis Coverage Award for Covid reporting, and has been a contributing editor at Nautilus Magazine. In 2021, Zeldovich released her first book, The Other Dark Matter, published by the University of Chicago Press, about the science and business of turning waste into wealth and health. You can find her on http://linazeldovich.com/ and @linazeldovich.