How Should Genetic Engineering Shape Our Future?
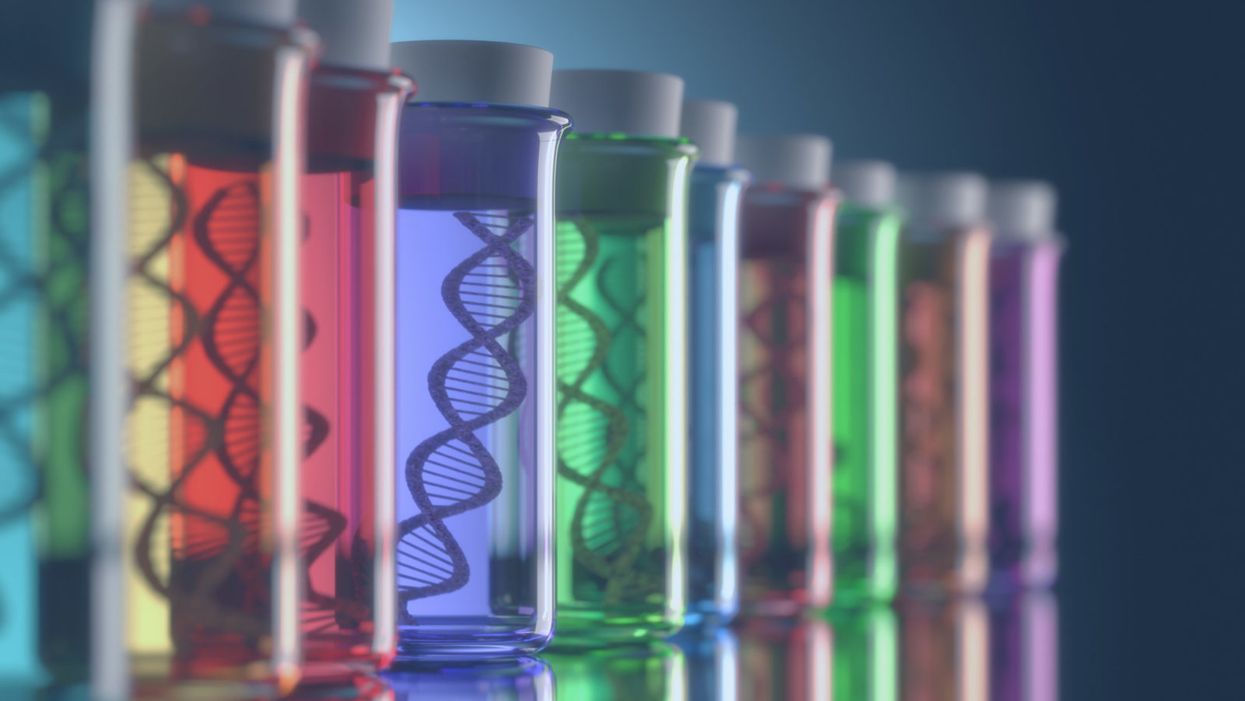
An artist's rendering of genetic codes.
Terror. Error. Success. These are the three outcomes that ethicists evaluating a new technology should fear. The possibility that a breakthrough might be used maliciously. The possibility that newly empowered scientists might make a catastrophic mistake. And the possibility that a technology will be so successful that it will change how we live in ways that we can only guess—and that we may not want.
These tools will allow scientists to practice genetic engineering on a scale that is simultaneously far more precise and far more ambitious than ever before.
It was true for the scientists behind the Manhattan Project, who bequeathed a fear of nuclear terror and nuclear error, even as global security is ultimately defined by these weapons of mass destruction. It was true for the developers of the automobile, whose invention has been weaponized by terrorists and kills 3,400 people by accident each day, even as the more than 1 billion cars on the road today have utterly reshaped where we live and how we move. And it is true for the researchers behind the revolution in gene editing and writing.
Put simply, these tools will allow scientists to practice genetic engineering on a scale that is simultaneously far more precise and far more ambitious than ever before. Editing techniques like CRISPR enable exact genetic repairs through a simple cut and paste of DNA, while synthetic biologists aim to redo entire genomes through the writing and substitution of synthetic genes. The technologies are complementary, and they herald an era when the book of life will be not just readable, but rewritable. Food crops, endangered animals, even the human body itself—all will eventually be programmable.
The benefits are easy to imagine: more sustainable crops; cures for terminal genetic disorders; even an end to infertility. Also easy to picture are the ethical pitfalls as the negative images of those same benefits.
Terror is the most straightforward. States have sought to use biology as a weapon at least since invading armies flung the corpses of plague victims into besieged castles. The 1975 biological weapons convention banned—with general success—the research and production of offensive bioweapons, though a handful of lone terrorists and groups like the Oregon-based Rajneeshee cult have still carried out limited bioweapon attacks. Those incidents ultimately caused little death and damage, in part because medical science is mostly capable of defending us from those pathogens that are most easily weaponized. But gene editing and writing offers the chance to engineer germs that could be far more effective than anything nature could develop. Imagine a virus that combines the lethality of Ebola with the transmissibility of the common cold—and in the new world of biology, if you can imagine something, you will eventually be able to create it.
The benefits are easy to imagine: more sustainable crops; cures for terminal genetic disorders; even an end to infertility. Also easy to picture are the ethical pitfalls.
That's one reason why James Clapper, then the U.S. director of national intelligence, added gene editing to the list of threats posed by "weapons of mass destruction and proliferation" in 2016. But these new tools aren't merely dangerous in the wrong hands—they can also be dangerous in the right hands. The list of labs accidents involving lethal bugs is much longer than you'd want to know, at least if you're the sort of person who likes to sleep at night. The U.S. recently lifted a ban on research that works to make existing pathogens, like the H5N1 avian flu virus, more virulent and transmissible, often using new technologies like gene editing. Such work can help medicine better prepare for what nature might throw at us, but it could also make the consequences of a lab error far more catastrophic. There's also the possibility that the use of gene editing and writing in nature—say, by CRISPRing disease-carrying mosquitoes to make them sterile—could backfire in some unforeseen way. Add in the fact that the techniques behind gene editing and writing are becoming simpler and more automated with every year, and eventually millions of people will be capable—through terror or error—of unleashing something awful on the world.
The good news is that both the government and the researchers driving these technologies are increasingly aware of the risks of bioterror and error. One government program, the Functional Genomic and Computational Assessment of Threats (Fun GCAT), provides funding for scientists to scan genetic data looking for the "accidental or intentional creation of a biological threat." Those in the biotech industry know to keep an eye out for suspicious orders—say, a new customer who orders part of the sequence of the Ebola or smallpox virus. "With every invention there is a good use and a bad use," Emily Leproust, the CEO of the commercial DNA synthesis startup Twist Bioscience, said in a recent interview. "What we try hard to do is put in place as many systems as we can to maximize the good stuff, and minimize any negative impact."
But the greatest ethical challenges in gene editing and writing will arise not from malevolence or mistakes, but from success. Through a new technology called in vitro gametogenesis (IVG), scientists are learning how to turn adult human cells like a piece of skin into lab-made sperm and egg cells. That would be a huge breakthrough for the infertile, or for same-sex couples who want to conceive a child biologically related to both partners. It would also open the door to using gene editing to tinker with those lab-made embryos. At first interventions would address any obvious genetic disorders, but those same tools would likely allow the engineering of a child's intelligence, height and other characteristics. We might be morally repelled today by such an ability, as many scientists and ethicists were repelled by in-vitro fertilization (IVF) when it was introduced four decades ago. Yet more than a million babies in the U.S. have been born through IVF in the years since. Ethics can evolve along with technology.
These new technologies offer control over the code of life, but only we as a society can seize control over where these tools will take us.
Fertility is just one human institution that stands to be changed utterly by gene editing and writing, and it's a change we can at least imagine. As the new biology grows more ambitious, it will alter society in ways we can't begin to picture. Harvard's George Church and New York University's Jef Boeke are leading an effort called HGP-Write to create a completely synthetic human genome. While gene editing allows scientists to make small changes to the genome, the gene synthesis that Church and his collaborators are developing allows for total genetic rewrites. "It's a difference between editing a book and writing one," Church said in an interview earlier this year.
Church is already working on synthesizing organs that would be resistant to viruses, while other researchers like Harris Wang at Columbia University are experimenting with bioengineering mammalian cells to produce nutrients like amino acids that we currently need to get from food. The horizon is endless—and so are the ethical concerns of success. What if parents feel pressure to engineer their children just so they don't fall behind their IVG peers? What if only the rich are able to access synthetic biology technologies that could make them stronger, smarter and longer lived? Could inequality become encoded in the genome?
These are questions that are different from the terror and errors fears around biosecurity, because they ask us to think hard about what kind of future we want. To their credit, Church and his collaborators have engaged bioethicists from the start of their work, as have the pioneers behind CRISPR. But the challenges coming from successful gene editing and writing are too large to be outsourced to professional ethicists. These new technologies offer control over the code of life, but only we as a society can seize control over where these tools will take us.
Indigenous wisdom plus honeypot ants could provide new antibiotics
Indigenous people in Australia dig pits next to a honeypot colony. Scientists think the honey can be used to make new antimicrobial drugs.
For generations, the Indigenous Tjupan people of Australia enjoyed the sweet treat of honey made by honeypot ants. As a favorite pastime, entire families would go searching for the underground colonies, first spotting a worker ant and then tracing it to its home. The ants, which belong to the species called Camponotus inflatus, usually build their subterranean homes near the mulga trees, Acacia aneura. Having traced an ant to its tree, it would be the women who carefully dug a pit next to a colony, cautious not to destroy the entire structure. Once the ant chambers were exposed, the women would harvest a small amount to avoid devastating the colony’s stocks—and the family would share the treat.
The Tjupan people also knew that the honey had antimicrobial properties. “You could use it for a sore throat,” says Danny Ulrich, a member of the Tjupan nation. “You could also use it topically, on cuts and things like that.”
These hunts have become rarer, as many of the Tjupan people have moved away and, up until now, the exact antimicrobial properties of the ant honey remained unknown. But recently, scientists Andrew Dong and Kenya Fernandes from the University of Sydney, joined Ulrich, who runs the Honeypot Ants tours in Kalgoorlie, a city in Western Australia, on a honey-gathering expedition. Afterwards, they ran a series of experiments analyzing the honey’s antimicrobial activity—and confirmed that the Indigenous wisdom was true. The honey was effective against Staphylococcus aureus, a common pathogen responsible for sore throats, skin infections like boils and sores, and also sepsis, which can result in death. Moreover, the honey also worked against two species of fungi, Cryptococcus and Aspergillus, which can be pathogenic to humans, especially those with suppressed immune systems.
In the era of growing antibiotic resistance and the rising threat of pathogenic fungi, these findings may help scientists identify and make new antimicrobial compounds. “Natural products have been honed over thousands and millions of years by nature and evolution,” says Fernandes. “And some of them have complex and intricate properties that make them really important as potential new antibiotics. “
In an era of growing resistance to antibiotics and new threats of fungi infections, the latest findings about honeypot ants are helping scientists identify new antimicrobial drugs.
Danny Ulrich
Bee honey is also known for its antimicrobial properties, but bees produce it very differently than the ants. Bees collect nectar from flowers, which they regurgitate at the hive and pack into the hexagonal honeycombs they build for storage. As they do so, they also add into the mix an enzyme called glucose oxidase produced by their glands. The enzyme converts atmospheric oxygen into hydrogen peroxide, a reactive molecule that destroys bacteria and acts as a natural preservative. After the bees pack the honey into the honeycombs, they fan it with their wings to evaporate the water. Once a honeycomb is full, the bees put a beeswax cover on it, where it stays well-preserved thanks to the enzymatic action, until the bees need it.
Less is known about the chemistry of ants’ honey-making. Similarly to bees, they collect nectar. They also collect the sweet sap of the mulga tree. Additionally, they also “milk” the aphids—small sap-sucking insects that live on the tree. When ants tickle the aphids with their antennae, the latter release a sweet substance, which the former also transfer to their colonies. That’s where the honey management difference becomes really pronounced. The ants don’t build any kind of structures to store their honey. Instead, they store it in themselves.
The workers feed their harvest to their fellow ants called repletes, stuffing them up to the point that their swollen bellies outgrow the ants themselves, looking like amber-colored honeypots—hence the name. Because of their size, repletes don’t move, but hang down from the chamber’s ceiling, acting as living feedstocks. When food becomes scarce, they regurgitate their reserves to their colony’s brethren. It’s not clear whether the repletes die afterwards or can be restuffed again. “That's a good question,” Dong says. “After they've been stretched, they can't really return to exactly the same shape.”
These replete ants are the “treat” the Tjupan women dug for. Once they saw the round-belly ants inside the chambers, they would reach in carefully and get a few scoops of them. “You see a lot of honeypot ants just hanging on the roof of the little openings,” says Ulrich’s mother, Edie Ulrich. The women would share the ants with family members who would eat them one by one. “They're very delicate,” shares Edie Ulrich—you have to take them out carefully, so they don’t accidentally pop and become a wasted resource. “Because you’d lose all this precious honey.”
Dong stumbled upon the honeypot ants phenomenon because he was interested in Indigenous foods and went on Ulrich’s tour. He quickly became fascinated with the insects and their role in the Indigenous culture. “The honeypot ants are culturally revered by the Indigenous people,” he says. Eventually he decided to test out the honey’s medicinal qualities.
The researchers were surprised to see that even the smallest, eight percent concentration of honey was able to arrest the growth of S. aureus.
To do this, the two scientists first diluted the ant honey with water. “We used something called doubling dilutions, which means that we made 32 percent dilutions, and then we halve that to 16 percent and then we half that to eight percent,” explains Fernandes. The goal was to obtain as much results as possible with the meager honey they had. “We had very, very little of the honeypot ant honey so we wanted to maximize the spectrum of results we can get without wasting too much of the sample.”
After that, the researchers grew different microbes inside a nutrient rich broth. They added the broth to the different honey dilutions and incubated the mixes for a day or two at the temperature favorable to the germs’ growth. If the resulting solution turned turbid, it was a sign that the bugs proliferated. If it stayed clear, it meant that the honey destroyed them. The researchers were surprised to see that even the smallest, eight percent concentration of honey was able to arrest the growth of S. aureus. “It was really quite amazing,” Fernandes says. “Eight milliliters of honey in 92 milliliters of water is a really tiny amount of honey compared to the amount of water.”
Similar to bee honey, the ants’ honey exhibited some peroxide antimicrobial activity, researchers found, but given how little peroxide was in the solution, they think the honey also kills germs by a different mechanism. “When we measured, we found that [the solution] did have some hydrogen peroxide, but it didn't have as much of it as we would expect based on how active it was,” Fernandes says. “Whether this hydrogen peroxide also comes from glucose oxidase or whether it's produced by another source, we don't really know,” she adds. The research team does have some hypotheses about the identity of this other germ-killing agent. “We think it is most likely some kind of antimicrobial peptide that is actually coming from the ant itself.”
The honey also has a very strong activity against the two types of fungi, Cryptococcus and Aspergillus. Both fungi are associated with trees and decaying leaves, as well as in the soils where ants live, so the insects likely have evolved some natural defense compounds, which end up inside the honey.
It wouldn’t be the first time when modern medicines take their origin from the natural world or from the indigenous people’s knowledge. The bark of the cinchona tree native to South America contains quinine, a substance that treats malaria. The Indigenous people of the Andes used the bark to quell fever and chills for generations, and when Europeans began to fall ill with malaria in the Amazon rainforest, they learned to use that medicine from the Andean people.
The wonder drug aspirin similarly takes its origin from a bark of a tree—in this case a willow.
Even some anticancer compounds originated from nature. A chemotherapy drug called Paclitaxel, was originally extracted from the Pacific yew trees, Taxus brevifolia. The samples of the Pacific yew bark were first collected in 1962 by researchers from the United States Department of Agriculture who were looking for natural compounds that might have anti-tumor activity. In December 1992, the FDA approved Paclitaxel (brand name Taxol) for the treatment of ovarian cancer and two years later for breast cancer.
In the era when the world is struggling to find new medicines fast enough to subvert a fungal or bacterial pandemic, these discoveries can pave the way to new therapeutics. “I think it's really important to listen to indigenous cultures and to take their knowledge because they have been using these sources for a really, really long time,” Fernandes says. Now we know it works, so science can elucidate the molecular mechanisms behind it, she adds. “And maybe it can even provide a lead for us to develop some kind of new treatments in the future.”
Lina Zeldovich has written about science, medicine and technology for Popular Science, Smithsonian, National Geographic, Scientific American, Reader’s Digest, the New York Times and other major national and international publications. A Columbia J-School alumna, she has won several awards for her stories, including the ASJA Crisis Coverage Award for Covid reporting, and has been a contributing editor at Nautilus Magazine. In 2021, Zeldovich released her first book, The Other Dark Matter, published by the University of Chicago Press, about the science and business of turning waste into wealth and health. You can find her on http://linazeldovich.com/ and @linazeldovich.
Blood Test Can Detect Lymphoma Cells Before a Tumor Grows Back
David Kurtz making DNA sequencing libraries in his lab.
When David M. Kurtz was doing his clinical fellowship at Stanford University Medical Center in 2009, specializing in lymphoma treatments, he found himself grappling with a question no one could answer. A typical regimen for these blood cancers prescribed six cycles of chemotherapy, but no one knew why. "The number seemed to be drawn out of a hat," Kurtz says. Some patients felt much better after just two doses, but had to endure the toxic effects of the entire course. For some elderly patients, the side effects of chemo are so harsh, they alone can kill. Others appeared to be cancer-free on the CT scans after the requisite six but then succumbed to it months later.
"Anecdotally, one patient decided to stop therapy after one dose because he felt it was so toxic that he opted for hospice instead," says Kurtz, now an oncologist at the center. "Five years down the road, he was alive and well. For him, just one dose was enough." Others would return for their one-year check up and find that their tumors grew back. Kurtz felt that while CT scans and MRIs were powerful tools, they weren't perfect ones. They couldn't tell him if there were any cancer cells left, stealthily waiting to germinate again. The scans only showed the tumor once it was back.
Blood cancers claim about 68,000 people a year, with a new diagnosis made about every three minutes, according to the Leukemia Research Foundation. For patients with B-cell lymphoma, which Kurtz focuses on, the survival chances are better than for some others. About 60 percent are cured, but the remaining 40 percent will relapse—possibly because they will have a negative CT scan, but still harbor malignant cells. "You can't see this on imaging," says Michael Green, who also treats blood cancers at University of Texas MD Anderson Medical Center.
The new blood test is sensitive enough to spot one cancerous perpetrator amongst one million other DNA molecules.
Kurtz wanted a better diagnostic tool, so he started working on a blood test that could capture the circulating tumor DNA or ctDNA. For that, he needed to identify the specific mutations typical for B-cell lymphomas. Working together with another fellow PhD student Jake Chabon, Kurtz finally zeroed-in on the tumor's genetic "appearance" in 2017—a pair of specific mutations sitting in close proximity to each other—a rare and telling sign. The human genome contains about 3 billion base pairs of nucleotides—molecules that compose genes—and in case of the B-cell lymphoma cells these two mutations were only a few base pairs apart. "That was the moment when the light bulb went on," Kurtz says.
The duo formed a company named Foresight Diagnostics, focusing on taking the blood test to the clinic. But knowing the tumor's mutational signature was only half the process. The other was fishing the tumor's DNA out of patients' bloodstream that contains millions of other DNA molecules, explains Chabon, now Foresight's CEO. It would be like looking for an escaped criminal in a large crowd. Kurtz and Chabon solved the problem by taking the tumor's "mug shot" first. Doctors would take the biopsy pre-treatment and sequence the tumor, as if taking the criminal's photo. After treatments, they would match the "mug shot" to all DNA molecules derived from the patient's blood sample to see if any molecular criminals managed to escape the chemo.
Foresight isn't the only company working on blood-based tumor detection tests, which are dubbed liquid biopsies—other companies such as Natera or ArcherDx developed their own. But in a recent study, the Foresight team showed that their method is significantly more sensitive in "fishing out" the cancer molecules than existing tests. Chabon says that this test can detect circulating tumor DNA in concentrations that are nearly 100 times lower than other methods. Put another way, it's sensitive enough to spot one cancerous perpetrator amongst one million other DNA molecules.
They also aim to extend their test to detect other malignancies such as lung, breast or colorectal cancers.
"It increases the sensitivity of detection and really catches most patients who are going to progress," says Green, the University of Texas oncologist who wasn't involved in the study, but is familiar with the method. It would also allow monitoring patients during treatment and making better-informed decisions about which therapy regimens would be most effective. "It's a minimally invasive test," Green says, and "it gives you a very high confidence about what's going on."
Having shown that the test works well, Kurtz and Chabon are planning a new trial in which oncologists would rely on their method to decide when to stop or continue chemo. They also aim to extend their test to detect other malignancies such as lung, breast or colorectal cancers. The latest genome sequencing technologies have sequenced and catalogued over 2,500 different tumor specimens and the Foresight team is analyzing this data, says Chabon, which gives the team the opportunity to create more molecular "mug shots."
The team hopes that that their blood cancer test will become available to patients within about five years, making doctors' job easier, and not only at the biological level. "When I tell patients, "good news, your cancer is in remission', they ask me, 'does it mean I'm cured?'" Kurtz says. "Right now I can't answer this question because I don't know—but I would like to." His company's test, he hopes, will enable him to reply with certainty. He'd very much like to have the power of that foresight.
This article is republished from our archives to coincide with Blood Cancer Awareness Month, which highlights progress in cancer diagnostics and treatment.
Lina Zeldovich has written about science, medicine and technology for Popular Science, Smithsonian, National Geographic, Scientific American, Reader’s Digest, the New York Times and other major national and international publications. A Columbia J-School alumna, she has won several awards for her stories, including the ASJA Crisis Coverage Award for Covid reporting, and has been a contributing editor at Nautilus Magazine. In 2021, Zeldovich released her first book, The Other Dark Matter, published by the University of Chicago Press, about the science and business of turning waste into wealth and health. You can find her on http://linazeldovich.com/ and @linazeldovich.