Scientists are making machines, wearable and implantable, to act as kidneys
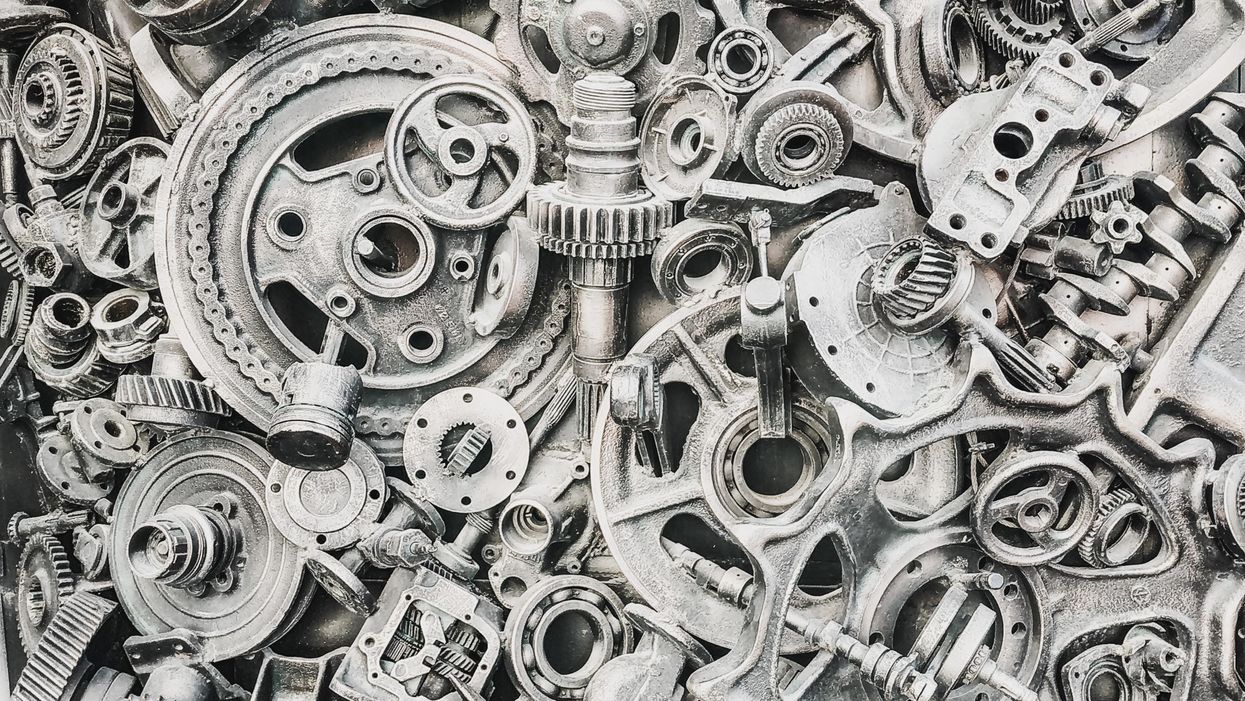
Recent advancements in engineering mean that the first preclinical trials for an artificial kidney could happen soon.
Like all those whose kidneys have failed, Scott Burton’s life revolves around dialysis. For nearly two decades, Burton has been hooked up (or, since 2020, has hooked himself up at home) to a dialysis machine that performs the job his kidneys normally would. The process is arduous, time-consuming, and expensive. Except for a brief window before his body rejected a kidney transplant, Burton has depended on machines to take the place of his kidneys since he was 12-years-old. His whole life, the 39-year-old says, revolves around dialysis.
“Whenever I try to plan anything, I also have to plan my dialysis,” says Burton says, who works as a freelance videographer and editor. “It’s a full-time job in itself.”
Many of those on dialysis are in line for a kidney transplant that would allow them to trade thrice-weekly dialysis and strict dietary limits for a lifetime of immunosuppressants. Burton’s previous transplant means that his body will likely reject another donated kidney unless it matches perfectly—something he’s not counting on. It’s why he’s enthusiastic about the development of artificial kidneys, small wearable or implantable devices that would do the job of a healthy kidney while giving users like Burton more flexibility for traveling, working, and more.
Still, the devices aren’t ready for testing in humans—yet. But recent advancements in engineering mean that the first preclinical trials for an artificial kidney could happen soon, according to Jonathan Himmelfarb, a nephrologist at the University of Washington.
“It would liberate people with kidney failure,” Himmelfarb says.
An engineering marvel
Compared to the heart or the brain, the kidney doesn’t get as much respect from the medical profession, but its job is far more complex. “It does hundreds of different things,” says UCLA’s Ira Kurtz.
Kurtz would know. He’s worked as a nephrologist for 37 years, devoting his career to helping those with kidney disease. While his colleagues in cardiology and endocrinology have seen major advances in the development of artificial hearts and insulin pumps, little has changed for patients on hemodialysis. The machines remain bulky and require large volumes of a liquid called dialysate to remove toxins from a patient’s blood, along with gallons of purified water. A kidney transplant is the next best thing to someone’s own, functioning organ, but with over 600,000 Americans on dialysis and only about 100,000 kidney transplants each year, most of those in kidney failure are stuck on dialysis.
Part of the lack of progress in artificial kidney design is the sheer complexity of the kidney’s job. Each of the 45 different cell types in the kidney do something different.
Part of the lack of progress in artificial kidney design is the sheer complexity of the kidney’s job. To build an artificial heart, Kurtz says, you basically need to engineer a pump. An artificial pancreas needs to balance blood sugar levels with insulin secretion. While neither of these tasks is simple, they are fairly straightforward. The kidney, on the other hand, does more than get rid of waste products like urea and other toxins. Each of the 45 different cell types in the kidney do something different, helping to regulate electrolytes like sodium, potassium, and phosphorous; maintaining blood pressure and water balance; guiding the body’s hormonal and inflammatory responses; and aiding in the formation of red blood cells.
There's been little progress for patients during Ira Kurtz's 37 years as a nephrologist. Artificial kidneys would change that.
UCLA
Dialysis primarily filters waste, and does so well enough to keep someone alive, but it isn’t a true artificial kidney because it doesn’t perform the kidney’s other jobs, according to Kurtz, such as sensing levels of toxins, wastes, and electrolytes in the blood. Due to the size and water requirements of existing dialysis machines, the equipment isn’t portable. Physicians write a prescription for a certain duration of dialysis and assess how well it’s working with semi-regular blood tests. The process of dialysis itself, however, is conducted blind. Doctors can’t tell how much dialysis a patient needs based on kidney values at the time of treatment, says Meera Harhay, a nephrologist at Drexel University in Philadelphia.
But it’s the impact of dialysis on their day-to-day lives that creates the most problems for patients. Only one-quarter of those on dialysis are able to remain employed (compared to 85% of similar-aged adults), and many report a low quality of life. Having more flexibility in life would make a major different to her patients, Harhay says.
“Almost half their week is taken up by the burden of their treatment. It really eats away at their freedom and their ability to do things that add value to their life,” she says.
Art imitates life
The challenge for artificial kidney designers was how to compress the kidney’s natural functions into a portable, wearable, or implantable device that wouldn’t need constant access to gallons of purified and sterilized water. The other universal challenge they faced was ensuring that any part of the artificial kidney that would come in contact with blood was kept germ-free to prevent infection.
As part of the 2021 KidneyX Prize, a partnership between the U.S. Department of Health and Human Services and the American Society of Nephrology, inventors were challenged to create prototypes for artificial kidneys. Himmelfarb’s team at the University of Washington’s Center for Dialysis Innovation won the prize by focusing on miniaturizing existing technologies to create a portable dialysis machine. The backpack sized AKTIV device (Ambulatory Kidney to Increase Vitality) will recycle dialysate in a closed loop system that removes urea from blood and uses light-based chemical reactions to convert the urea to nitrogen and carbon dioxide, which allows the dialysate to be recirculated.
Himmelfarb says that the AKTIV can be used when at home, work, or traveling, which will give users more flexibility and freedom. “If you had a 30-pound device that you could put in the overhead bins when traveling, you could go visit your grandkids,” he says.
Kurtz’s team at UCLA partnered with the U.S. Kidney Research Corporation and Arkansas University to develop a dialysate-free desktop device (about the size of a small printer) as the first phase of a progression that will he hopes will lead to something small and implantable. Part of the reason for the artificial kidney’s size, Kurtz says, is the number of functions his team are cramming into it. Not only will it filter urea from blood, but it will also use electricity to help regulate electrolyte levels in a process called electrodeionization. Kurtz emphasizes that these additional functions are what makes his design a true artificial kidney instead of just a small dialysis machine.
One version of an artificial kidney.
UCLA
“It doesn't have just a static function. It has a bank of sensors that measure chemicals in the blood and feeds that information back to the device,” Kurtz says.
Other startups are getting in on the game. Nephria Bio, a spinout from the South Korean-based EOFlow, is working to develop a wearable dialysis device, akin to an insulin pump, that uses miniature cartridges with nanomaterial filters to clean blood (Harhay is a scientific advisor to Nephria). Ian Welsford, Nephria’s co-founder and CTO, says that the device’s design means that it can also be used to treat acute kidney injuries in resource-limited settings. These potentials have garnered interest and investment in artificial kidneys from the U.S. Department of Defense.
For his part, Burton is most interested in an implantable device, as that would give him the most freedom. Even having a regular outpatient procedure to change batteries or filters would be a minor inconvenience to him.
“Being plugged into a machine, that’s not mimicking life,” he says.
This article was first published by Leaps.org on May 5, 2022.
A mosquito under the microscope.
Two years, six million deaths and still counting, scientists are searching for answers to prevent another COVID-19-like tragedy from ever occurring again. And it’s a gargantuan task.
Our disturbed ecosystems are creating more favorable conditions for the spread of infectious disease. Global warming, deforestation, rising sea levels and flooding have contributed to a rise in mosquito-borne infections and longer tick seasons. Disease-carrying animals are in closer range to other species and humans as they migrate to escape the heat. Bats are thought to have carried the SARS-CoV-2 virus to Wuhan, either directly or through another host animal, but thousands of novel viruses are lurking within other wild creatures.
Understanding how climate change contributes to the spread of disease is critical in predicting and thwarting future calamities. But the problem is that predictive models aren’t yet where they need to be for forecasting with certainty beyond the next year, as we could for weather, for instance.
The association between climate and infectious disease is poorly understood, says Irina Tezaur, a computational scientist at Sandia National Laboratories. “Correlations have been observed but it’s not known if these correlations translate to causal relationships.”
To make accurate longer-term predictions, scientists need more empirical data, multiple datasets specific to locations and diseases, and the ability to calculate risks that depend on unpredictable nature and human behavior. Another obstacle is that climate scientists and epidemiologists are not collaborating effectively, so some researchers are calling for a multidisciplinary approach, a new field called Outbreak Science.
Climate scientists are far ahead of epidemiologists in gathering essential data.
Earth System Models—combining the interactions of atmosphere, ocean, land, ice and biosphere—have been in place for two decades to monitor the effects of global climate change. These models must be combined with epidemiological and human model research, areas that are easily skewed by unpredictable elements, from extreme weather events to public environmental policy shifts.
“There is never just one driver in tracking the impact of climate on infectious disease,” says Joacim Rocklöv, a professor at the Heidelberg Institute of Global Health & Heidelberg Interdisciplinary Centre for Scientific Computing in Germany. Rocklöv has studied how climate affects vector-borne diseases—those transmitted to humans by mosquitoes, ticks or fleas. “You need to disentangle the variables to find out how much difference climate makes to the outcome and how much is other factors.” Determinants from deforestation to population density to lack of healthcare access influence the spread of disease.
Even though climate change is not the primary driver of infectious disease today, it poses a major threat to public health in the future, says Rocklöv.
The promise of predictive modeling
“Models are simplifications of a system we’re trying to understand,” says Jeremy Hess, who directs the Center for Health and the Global Environment at University of Washington in Seattle. “They’re tools for learning that improve over time with new observations.”
Accurate predictions depend on high-quality, long-term observational data but models must start with assumptions. “It’s not possible to apply an evidence-based approach for the next 40 years,” says Rocklöv. “Using models to experiment and learn is the only way to figure out what climate means for infectious disease. We collect data and analyze what already happened. What we do today will not make a difference for several decades.”
To improve accuracy, scientists develop and draw on thousands of models to cover as many scenarios as possible. One model may capture the dynamics of disease transmission while another focuses on immunity data or ocean influences or seasonal components of a virus. Further, each model needs to be disease-specific and often location-specific to be useful.
“All models have biases so it’s important to use a suite of models,” Tezaur stresses.
The modeling scientist chooses the drivers of change and parameters based on the question explored. The drivers could be increased precipitation, poverty or mosquito prevalence, for instance. Later, the scientist may need to isolate the effect of one driver so that will require another model.
There have been some related successes, such as the latest models for mosquito-borne diseases like Dengue, Zika and malaria as well as those for flu and tick-borne diseases, says Hess.
Rocklöv was part of a research team that used test data from 2018 and 2019 to identify regions at risk for West Nile virus outbreaks. Using AI, scientists were able to forecast outbreaks of the virus for the entire transmission season in Europe. “In the end, we want data-driven models; that’s what AI can accomplish,” says Rocklöv. Other researchers are making an important headway in creating a framework to predict novel host–parasite interactions.
Modeling studies can run months, years or decades. “The scientist is working with layers of data. The challenge is how to transform and couple different models together on a planetary scale,” says Jeanne Fair, a scientist at Los Alamos National Laboratory, Biosecurity and Public Health, in New Mexico.
Disease forecasting will require a significant investment into the infrastructure needed to collect data about the environment, vectors, and hosts a tall spatial and temporal resolutions.
And it’s a constantly changing picture. A modeling study in an April 2022 issue of Nature predicted that thousands of animals will migrate to cooler locales as temperatures rise. This means that various species will come into closer contact with people and other mammals for the first time. This is likely to increase the risk of emerging infectious disease transmitted from animals to humans, especially in Africa and Asia.
Other things can happen too. Global warming could precipitate viral mutations or new infectious diseases that don’t respond to antimicrobial treatments. Insecticide-resistant mosquitoes could evolve. Weather-related food insecurity could increase malnutrition and weaken people’s immune systems. And the impact of an epidemic will be worse if it co-occurs during a heatwave, flood, or drought, says Hess.
The devil is in the climate variables
Solid predictions about the future of climate and disease are not possible with so many uncertainties. Difficult-to-measure drivers must be added to the empirical model mix, such as land and water use, ecosystem changes or the public’s willingness to accept a vaccine or practice social distancing. Nor is there any precedent for calculating the effect of climate changes that are accelerating at a faster speed than ever before.
The most critical climate variables thought to influence disease spread are temperature, precipitation, humidity, sunshine and wind, according to Tezaur’s research. And then there are variables within variables. Influenza scientists, for example, found that warm winters were predictors of the most severe flu seasons in the following year.
The human factor may be the most challenging determinant. To what degree will people curtail greenhouse gas emissions, if at all? The swift development of effective COVID-19 vaccines was a game-changer, but will scientists be able to repeat it during the next pandemic? Plus, no model could predict the amount of internet-fueled COVID-19 misinformation, Fair noted. To tackle this issue, infectious disease teams are looking to include more sociologists and political scientists in their modeling.
Addressing the gaps
Currently, researchers are focusing on the near future, predicting for next year, says Fair. “When it comes to long-term, that’s where we have the most work to do.” While scientists cannot foresee how political influences and misinformation spread will affect models, they are positioned to make headway in collecting and assessing new data streams that have never been merged.
Disease forecasting will require a significant investment into the infrastructure needed to collect data about the environment, vectors, and hosts at all spatial and temporal resolutions, Fair and her co-authors stated in their recent study. For example real-time data on mosquito prevalence and diversity in various settings and times is limited or non-existent. Fair also would like to see standards set in mosquito data collection in every country. “Standardizing across the US would be a huge accomplishment,” she says.
Understanding how climate change contributes to the spread of disease is critical for thwarting future calamities.
Jeanne Fair
Hess points to a dearth of data in local and regional datasets about how extreme weather events play out in different geographic locations. His research indicates that Africa and the Middle East experienced substantial climate shifts, for example, but are unrepresented in the evidentiary database, which limits conclusions. “A model for dengue may be good in Singapore but not necessarily in Port-au-Prince,” Hess explains. And, he adds, scientists need a way of evaluating models for how effective they are.
The hope, Rocklöv says, is that in the future we will have data-driven models rather than theoretical ones. In turn, sharper statistical analyses can inform resource allocation and intervention strategies to prevent outbreaks.
Most of all, experts emphasize that epidemiologists and climate scientists must stop working in silos. If scientists can successfully merge epidemiological data with climatic, biological, environmental, ecological and demographic data, they will make better predictions about complex disease patterns. Modeling “cross talk” and among disciplines and, in some cases, refusal to release data between countries is hindering discovery and advances.
It’s time for bold transdisciplinary action, says Hess. He points to initiatives that need funding in disease surveillance and control; developing and testing interventions; community education and social mobilization; decision-support analytics to predict when and where infections will emerge; advanced methodologies to improve modeling; training scientists in data management and integrated surveillance.
Establishing a new field of Outbreak Science to coordinate collaboration would accelerate progress. Investment in decision-support modeling tools for public health teams, policy makers, and other long-term planning stakeholders is imperative, too. We need to invest in programs that encourage people from climate modeling and epidemiology to work together in a cohesive fashion, says Tezaur. Joining forces is the only way to solve the formidable challenges ahead.
This article originally appeared in One Health/One Planet, a single-issue magazine that explores how climate change and other environmental shifts are increasing vulnerabilities to infectious diseases by land and by sea. The magazine probes how scientists are making progress with leaders in other fields toward solutions that embrace diverse perspectives and the interconnectedness of all lifeforms and the planet.
Scientists use AI to predict how hospital stays will go
In this week's Friday Five, research on the best time to wrap up eating for the night, how to use AI to predict how hospital stays will go, a new way to armor the shields of our livers against cancer, super neurons in super agers - and much more.
The Friday Five covers five stories in research that you may have missed this week. There are plenty of controversies and troubling ethical issues in science – and we get into many of them in our online magazine – but this news roundup focuses on scientific creativity and progress to give you a therapeutic dose of inspiration headed into the weekend.
Here are the promising studies covered in this week's Friday Five:
- The problem with bedtime munching
- Scientists use AI to predict how stays in hospitals will go
- How to armor the shields of our livers against cancer
- One big step to save the world: turn one kind of plastic into another
- The perfect recipe for tiny brains
And an honorable mention this week: Bigger is better when it comes to super neurons in super agers