Scientists are making machines, wearable and implantable, to act as kidneys
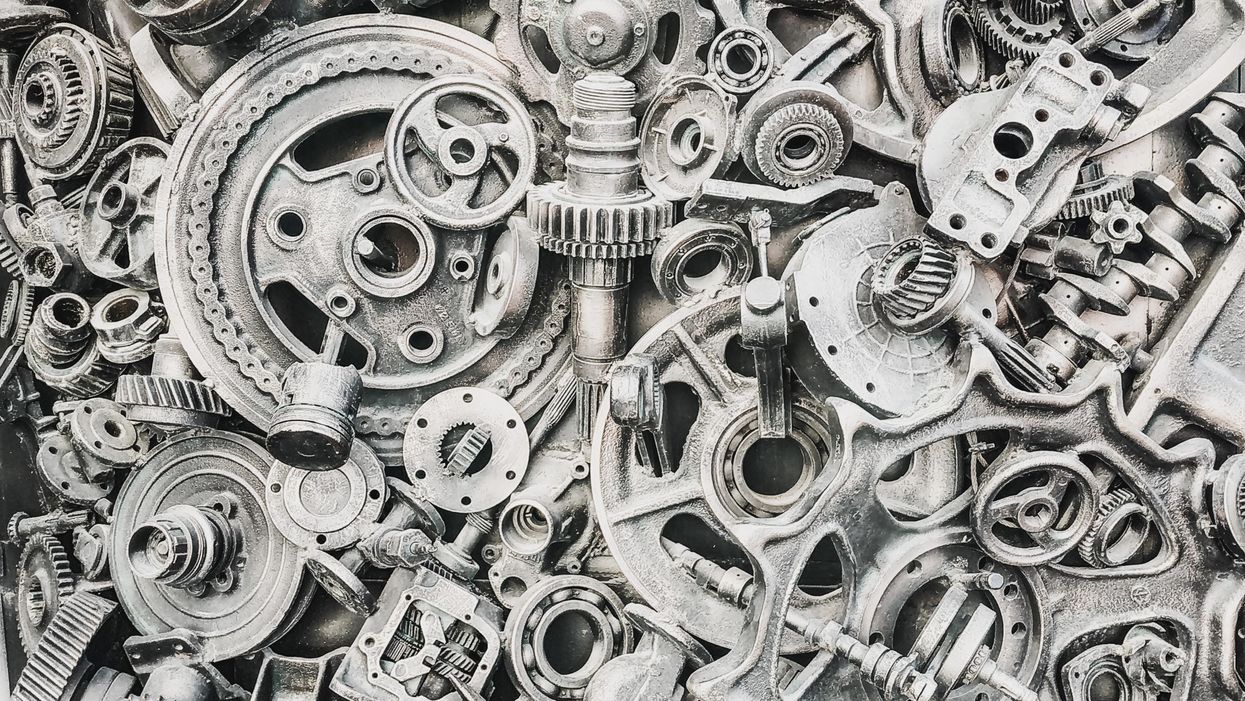
Recent advancements in engineering mean that the first preclinical trials for an artificial kidney could happen soon.
Like all those whose kidneys have failed, Scott Burton’s life revolves around dialysis. For nearly two decades, Burton has been hooked up (or, since 2020, has hooked himself up at home) to a dialysis machine that performs the job his kidneys normally would. The process is arduous, time-consuming, and expensive. Except for a brief window before his body rejected a kidney transplant, Burton has depended on machines to take the place of his kidneys since he was 12-years-old. His whole life, the 39-year-old says, revolves around dialysis.
“Whenever I try to plan anything, I also have to plan my dialysis,” says Burton says, who works as a freelance videographer and editor. “It’s a full-time job in itself.”
Many of those on dialysis are in line for a kidney transplant that would allow them to trade thrice-weekly dialysis and strict dietary limits for a lifetime of immunosuppressants. Burton’s previous transplant means that his body will likely reject another donated kidney unless it matches perfectly—something he’s not counting on. It’s why he’s enthusiastic about the development of artificial kidneys, small wearable or implantable devices that would do the job of a healthy kidney while giving users like Burton more flexibility for traveling, working, and more.
Still, the devices aren’t ready for testing in humans—yet. But recent advancements in engineering mean that the first preclinical trials for an artificial kidney could happen soon, according to Jonathan Himmelfarb, a nephrologist at the University of Washington.
“It would liberate people with kidney failure,” Himmelfarb says.
An engineering marvel
Compared to the heart or the brain, the kidney doesn’t get as much respect from the medical profession, but its job is far more complex. “It does hundreds of different things,” says UCLA’s Ira Kurtz.
Kurtz would know. He’s worked as a nephrologist for 37 years, devoting his career to helping those with kidney disease. While his colleagues in cardiology and endocrinology have seen major advances in the development of artificial hearts and insulin pumps, little has changed for patients on hemodialysis. The machines remain bulky and require large volumes of a liquid called dialysate to remove toxins from a patient’s blood, along with gallons of purified water. A kidney transplant is the next best thing to someone’s own, functioning organ, but with over 600,000 Americans on dialysis and only about 100,000 kidney transplants each year, most of those in kidney failure are stuck on dialysis.
Part of the lack of progress in artificial kidney design is the sheer complexity of the kidney’s job. Each of the 45 different cell types in the kidney do something different.
Part of the lack of progress in artificial kidney design is the sheer complexity of the kidney’s job. To build an artificial heart, Kurtz says, you basically need to engineer a pump. An artificial pancreas needs to balance blood sugar levels with insulin secretion. While neither of these tasks is simple, they are fairly straightforward. The kidney, on the other hand, does more than get rid of waste products like urea and other toxins. Each of the 45 different cell types in the kidney do something different, helping to regulate electrolytes like sodium, potassium, and phosphorous; maintaining blood pressure and water balance; guiding the body’s hormonal and inflammatory responses; and aiding in the formation of red blood cells.
There's been little progress for patients during Ira Kurtz's 37 years as a nephrologist. Artificial kidneys would change that.
UCLA
Dialysis primarily filters waste, and does so well enough to keep someone alive, but it isn’t a true artificial kidney because it doesn’t perform the kidney’s other jobs, according to Kurtz, such as sensing levels of toxins, wastes, and electrolytes in the blood. Due to the size and water requirements of existing dialysis machines, the equipment isn’t portable. Physicians write a prescription for a certain duration of dialysis and assess how well it’s working with semi-regular blood tests. The process of dialysis itself, however, is conducted blind. Doctors can’t tell how much dialysis a patient needs based on kidney values at the time of treatment, says Meera Harhay, a nephrologist at Drexel University in Philadelphia.
But it’s the impact of dialysis on their day-to-day lives that creates the most problems for patients. Only one-quarter of those on dialysis are able to remain employed (compared to 85% of similar-aged adults), and many report a low quality of life. Having more flexibility in life would make a major different to her patients, Harhay says.
“Almost half their week is taken up by the burden of their treatment. It really eats away at their freedom and their ability to do things that add value to their life,” she says.
Art imitates life
The challenge for artificial kidney designers was how to compress the kidney’s natural functions into a portable, wearable, or implantable device that wouldn’t need constant access to gallons of purified and sterilized water. The other universal challenge they faced was ensuring that any part of the artificial kidney that would come in contact with blood was kept germ-free to prevent infection.
As part of the 2021 KidneyX Prize, a partnership between the U.S. Department of Health and Human Services and the American Society of Nephrology, inventors were challenged to create prototypes for artificial kidneys. Himmelfarb’s team at the University of Washington’s Center for Dialysis Innovation won the prize by focusing on miniaturizing existing technologies to create a portable dialysis machine. The backpack sized AKTIV device (Ambulatory Kidney to Increase Vitality) will recycle dialysate in a closed loop system that removes urea from blood and uses light-based chemical reactions to convert the urea to nitrogen and carbon dioxide, which allows the dialysate to be recirculated.
Himmelfarb says that the AKTIV can be used when at home, work, or traveling, which will give users more flexibility and freedom. “If you had a 30-pound device that you could put in the overhead bins when traveling, you could go visit your grandkids,” he says.
Kurtz’s team at UCLA partnered with the U.S. Kidney Research Corporation and Arkansas University to develop a dialysate-free desktop device (about the size of a small printer) as the first phase of a progression that will he hopes will lead to something small and implantable. Part of the reason for the artificial kidney’s size, Kurtz says, is the number of functions his team are cramming into it. Not only will it filter urea from blood, but it will also use electricity to help regulate electrolyte levels in a process called electrodeionization. Kurtz emphasizes that these additional functions are what makes his design a true artificial kidney instead of just a small dialysis machine.
One version of an artificial kidney.
UCLA
“It doesn't have just a static function. It has a bank of sensors that measure chemicals in the blood and feeds that information back to the device,” Kurtz says.
Other startups are getting in on the game. Nephria Bio, a spinout from the South Korean-based EOFlow, is working to develop a wearable dialysis device, akin to an insulin pump, that uses miniature cartridges with nanomaterial filters to clean blood (Harhay is a scientific advisor to Nephria). Ian Welsford, Nephria’s co-founder and CTO, says that the device’s design means that it can also be used to treat acute kidney injuries in resource-limited settings. These potentials have garnered interest and investment in artificial kidneys from the U.S. Department of Defense.
For his part, Burton is most interested in an implantable device, as that would give him the most freedom. Even having a regular outpatient procedure to change batteries or filters would be a minor inconvenience to him.
“Being plugged into a machine, that’s not mimicking life,” he says.
This article was first published by Leaps.org on May 5, 2022.
How a Deadly Fire Gave Birth to Modern Medicine
The Cocoanut Grove fire in Boston in 1942 tragically claimed 490 lives, but was the catalyst for several important medical advances.
On the evening of November 28, 1942, more than 1,000 revelers from the Boston College-Holy Cross football game jammed into the Cocoanut Grove, Boston's oldest nightclub. When a spark from faulty wiring accidently ignited an artificial palm tree, the packed nightspot, which was only designed to accommodate about 500 people, was quickly engulfed in flames. In the ensuing panic, hundreds of people were trapped inside, with most exit doors locked. Bodies piled up by the only open entrance, jamming the exits, and 490 people ultimately died in the worst fire in the country in forty years.
"People couldn't get out," says Dr. Kenneth Marshall, a retired plastic surgeon in Boston and president of the Cocoanut Grove Memorial Committee. "It was a tragedy of mammoth proportions."
Within a half an hour of the start of the blaze, the Red Cross mobilized more than five hundred volunteers in what one newspaper called a "Rehearsal for Possible Blitz." The mayor of Boston imposed martial law. More than 300 victims—many of whom subsequently died--were taken to Boston City Hospital in one hour, averaging one victim every eleven seconds, while Massachusetts General Hospital admitted 114 victims in two hours. In the hospitals, 220 victims clung precariously to life, in agonizing pain from massive burns, their bodies ravaged by infection.
The scene of the fire.
Boston Public Library
Tragic Losses Prompted Revolutionary Leaps
But there is a silver lining: this horrific disaster prompted dramatic changes in safety regulations to prevent another catastrophe of this magnitude and led to the development of medical techniques that eventually saved millions of lives. It transformed burn care treatment and the use of plasma on burn victims, but most importantly, it introduced to the public a new wonder drug that revolutionized medicine, midwifed the birth of the modern pharmaceutical industry, and nearly doubled life expectancy, from 48 years at the turn of the 20th century to 78 years in the post-World War II years.
The devastating grief of the survivors also led to the first published study of post-traumatic stress disorder by pioneering psychiatrist Alexandra Adler, daughter of famed Viennese psychoanalyst Alfred Adler, who was a student of Freud. Dr. Adler studied the anxiety and depression that followed this catastrophe, according to the New York Times, and "later applied her findings to the treatment World War II veterans."
Dr. Ken Marshall is intimately familiar with the lingering psychological trauma of enduring such a disaster. His mother, an Irish immigrant and a nurse in the surgical wards at Boston City Hospital, was on duty that cold Thanksgiving weekend night, and didn't come home for four days. "For years afterward, she'd wake up screaming in the middle of the night," recalls Dr. Marshall, who was four years old at the time. "Seeing all those bodies lined up in neat rows across the City Hospital's parking lot, still in their evening clothes. It was always on her mind and memories of the horrors plagued her for the rest of her life."
The sheer magnitude of casualties prompted overwhelmed physicians to try experimental new procedures that were later successfully used to treat thousands of battlefield casualties. Instead of cutting off blisters and using dyes and tannic acid to treat burned tissues, which can harden the skin, they applied gauze coated with petroleum jelly. Doctors also refined the formula for using plasma--the fluid portion of blood and a medical technology that was just four years old--to replenish bodily liquids that evaporated because of the loss of the protective covering of skin.
"Every war has given us a new medical advance. And penicillin was the great scientific advance of World War II."
"The initial insult with burns is a loss of fluids and patients can die of shock," says Dr. Ken Marshall. "The scientific progress that was made by the two institutions revolutionized fluid management and topical management of burn care forever."
Still, they could not halt the staph infections that kill most burn victims—which prompted the first civilian use of a miracle elixir that was being secretly developed in government-sponsored labs and that ultimately ushered in a new age in therapeutics. Military officials quickly realized this disaster could provide an excellent natural laboratory to test the effectiveness of this drug and see if it could be used to treat the acute traumas of combat in this unfortunate civilian approximation of battlefield conditions. At the time, the very existence of this wondrous medicine—penicillin—was a closely guarded military secret.
From Forgotten Lab Experiment to Wonder Drug
In 1928, Alexander Fleming discovered the curative powers of penicillin, which promised to eradicate infectious pathogens that killed millions every year. But the road to mass producing enough of the highly unstable mold was littered with seemingly unsurmountable obstacles and it remained a forgotten laboratory curiosity for over a decade. But Fleming never gave up and penicillin's eventual rescue from obscurity was a landmark in scientific history.
In 1940, a group at Oxford University, funded in part by the Rockefeller Foundation, isolated enough penicillin to test it on twenty-five mice, which had been infected with lethal doses of streptococci. Its therapeutic effects were miraculous—the untreated mice died within hours, while the treated ones played merrily in their cages, undisturbed. Subsequent tests on a handful of patients, who were brought back from the brink of death, confirmed that penicillin was indeed a wonder drug. But Britain was then being ravaged by the German Luftwaffe during the Blitz, and there were simply no resources to devote to penicillin during the Nazi onslaught.
In June of 1941, two of the Oxford researchers, Howard Florey and Ernst Chain, embarked on a clandestine mission to enlist American aid. Samples of the temperamental mold were stored in their coats. By October, the Roosevelt Administration had recruited four companies—Merck, Squibb, Pfizer and Lederle—to team up in a massive, top-secret development program. Merck, which had more experience with fermentation procedures, swiftly pulled away from the pack and every milligram they produced was zealously hoarded.
After the nightclub fire, the government ordered Merck to dispatch to Boston whatever supplies of penicillin that they could spare and to refine any crude penicillin broth brewing in Merck's fermentation vats. After working in round-the-clock relays over the course of three days, on the evening of December 1st, 1942, a refrigerated truck containing thirty-two liters of injectable penicillin left Merck's Rahway, New Jersey plant. It was accompanied by a convoy of police escorts through four states before arriving in the pre-dawn hours at Massachusetts General Hospital. Dozens of people were rescued from near-certain death in the first public demonstration of the powers of the antibiotic, and the existence of penicillin could no longer be kept secret from inquisitive reporters and an exultant public. The next day, the Boston Globe called it "priceless" and Time magazine dubbed it a "wonder drug."
Within fourteen months, penicillin production escalated exponentially, churning out enough to save the lives of thousands of soldiers, including many from the Normandy invasion. And in October 1945, just weeks after the Japanese surrender ended World War II, Alexander Fleming, Howard Florey and Ernst Chain were awarded the Nobel Prize in medicine. But penicillin didn't just save lives—it helped build some of the most innovative medical and scientific companies in history, including Merck, Pfizer, Glaxo and Sandoz.
"Every war has given us a new medical advance," concludes Marshall. "And penicillin was the great scientific advance of World War II."
This Boy Struggled to Walk Before Gene Therapy. Now, Such Treatments Are Poised to Explode.
Conner Curran, now 10 years old, can walk more than two miles after gene therapy treatment for his Duchenne's muscular dystrophy.
Conner Curran was diagnosed with Duchenne's muscular dystrophy in 2015 when he was four years old. It's the most severe form of the genetic disease, with a nearly inevitable progression toward total paralysis. Many Duchenne's patients die in their teens; the average lifespan is 26.
But Conner, who is now 10, has experienced some astonishing improvements in recent years. He can now walk for more than two miles at a time – an impossible journey when he was younger.
In 2018, Conner became the very first patient to receive gene therapy specific to treating Duchenne's. In the initial clinical trial of nine children, nearly 80 percent reacted positively to the treatment). A larger-scale stage 3 clinical trial is currently underway, with initial results expected next year.
Gene therapy involves altering the genes in an individual's cells to stop or treat a disease. Such a procedure may be performed by adding new gene material to existing cells, or editing the defective genes to improve their functionality.
That the medical world is on the cusp of a successful treatment for a crippling and deadly disease is the culmination of more than 35 years of work by Dr. Jude Samulski, a professor of pharmacology at the University of North Carolina School of Medicine in Chapel Hill. More recently, he's become a leading gene therapy entrepreneur.
But Samulski likens this breakthrough to the frustrations of solving a Rubik's cube. "Just because one side is now all the color yellow does not mean that it is completely aligned," he says.
Although Conner's life and future have dramatically improved, he's not cured. The gene therapy tamed but did not extinguish his disorder: Conner is now suffering from the equivalent of Becker's muscular dystrophy, a milder form of the disease with symptoms that appear later in life and progress more slowly. Moreover, the loss of muscle cells Conner suffered prior to the treatment is permanent.
"It will take more time and more innovations," Samulski says of finding an even more effective gene therapy for muscular dystrophy.
Conner's family is still overjoyed with the results. "Jude's grit and determination gave Conner a chance at a new life, one that was not in his cards before gene therapy," says his mother Jessica Curran. She adds that "Conner is more confident than before and enjoys life, even though he has limitations, if compared to his brothers or peers."
Conner Curran holding a football post gene therapy treatment.
Courtesy of the Curran family
For now, the use of gene therapy as a treatment for diseases and disorders remains relatively isolated. On paper at least, progress appears glacially slow. In 2018, there were four FDA-approved gene therapies (excluding those reliant on bone marrow/stem cell transplants or implants). Today, there are 10. One therapy is solely for the cosmetic purpose of reducing facial lines and folds.
Nevertheless, experts in the space believe gene therapy is poised to expand dramatically.
"Certainly in the next three to five years you will see dozens of gene therapies and cell therapies be approved," says Dr. Pavan Cheruvu, who is CEO of Sio Gene Therapies in New York. The company is developing treatments for Parkinson's disease and Tay-Sachs, among other diseases.
Cheruvu's conclusion is supported by NEWDIGS, a think tank at the Massachusetts Institute of Technology that keeps tabs on gene therapy developments. NEWDIGS predicts there will be at least 60 gene therapies approved for use in the U.S. by the end of the decade. That number could be closer to 100 if Chinese researchers and biotech ventures decide the American market is a good fit for the therapies they develop.
"We are watching something of a conditional evolution, like a dot-com, or cellphones that were sizes of shoeboxes that have now matured to the size of wafers. Our space will follow along very similarly."
Dr. Carsten Brunn, a chemist by training and CEO of Selecta Biosciences outside of Boston, is developing ways to reduce the immune responses in patients who receive gene therapy. He observes that there are more than 300 therapies in development and thousands of clinical trials underway. "It's definitely an exciting time in the field," he says.
That's a far cry from the environment of little more than a decade ago. Research and investment in gene therapy had been brought low for years after the death of teenager Jesse Gelsinger in 1999 while he had been enrolled in a clinical trial to treat a liver disease. Gene therapy was a completely novel concept back then, and his death created existential questions about whether it was a proper pathway to pursue. Cheruvu, a cardiologist, calls the years after Gelsinger's death an "ice age" for gene therapy.
However, those dark years eventually yielded to a thaw. And while there have been some recent stumbles, they are considered part of the trial-and-error that has often accompanied medical research as opposed to an ominous "stop" sign.
The deaths of three patients last year receiving gene therapy for myotubular myopathy – a degenerative disease that causes severe muscle weakness – promptly ended the clinical trial in which they were enrolled. However, the incident caused few ripples beyond that. Researchers linked the deaths to dosage sizes that caused liver toxicity, as opposed to the gene therapy itself being an automatic death sentence; younger patients who received lower doses due to a less advanced disease state experienced improvements.
The gene sequencing and editing that helped create vaccines for COVID-19 in record time also bolstered the argument for more investment in research and development. Cheruvu notes that the field has usually been the domain of investors with significant expertise in the field; these days, more money is flowing in from generalists.
The Challenges Ahead
What will be the next step in gene therapy's evolution? Many of Samulski's earliest innovations came in the laboratory, for example. Then that led to him forming a company called AskBio in collaboration with the Muscular Dystrophy Association. AskBio sold its gene therapy to Pfizer five years ago to assure that enough could be manufactured for stage 3 clinical trials and eventually reach the market.
Cheruvu suggests that many future gene therapy innovations will be the result of what he calls "congruent innovation." That means publicly funded laboratories and privately funded companies might develop treatments separately or in collaboration. Or, university scientists may depend on private ventures to solve one of gene therapy's most vexing issues: producing enough finished material to test and treat on a large scale. "Manufacturing is a real bottleneck right now," Brunn says.
The alternative is referred to in the sector as the "valley of death": a lab has found a promising treatment, but is not far enough along in development to submit an investigational new drug application with the FDA. The promise withers away as a result. But the new abundance of venture capital for gene therapy has made this scenario less of an issue for private firms, some of which have received hundreds of millions of dollars in funding.
There are also numerous clinical challenges. Many gene therapies use what are known as adeno-associated virus vectors (AAVs) to deliver treatments. They are hollowed-out husks of viruses that can cause a variety of mostly mild maladies ranging from colds to pink eye. They are modified to deliver the genetic material used in the therapy. Most of these vectors trigger an antibody reaction that limits treatments to a single does or a handful of smaller ones. That can limit the potential progress for patients – an issue referred to as treatment "durability."
Although vectors from animals such as horses trigger far less of an antibody reaction in patients -- and there has been significant work done on using artificial vectors -- both are likely years away from being used on a large scale. "For the foreseeable future, AAV is the delivery system of choice," Brunn says.
Also, there will likely be demand for concurrent gene therapies that can lead to a complete cure – not only halting the progress of Duchenne's in kids like Conner Curran, but regenerating their lost muscle cells, perhaps through some form of stem cell therapy or another treatment that has yet to be devised.
Nevertheless, Samulski believes demand for imperfect treatments will be high – particularly with a disease such as muscular dystrophy, where many patients are mere months from spending the remainder of their lives in wheelchairs. But Samulski believes those therapies will also inevitably evolve into something far more effective.
"We are watching something of a conditional evolution, like a dot-com, or cellphones that were sizes of shoeboxes that have now matured to the size of wafers," he says. "Our space will follow along very similarly."
Jessica Curran will remain forever grateful for what her son has received: "Jude gave us new hope. He gave us something that is priceless – a chance to watch Conner grow up and live out his own dreams."