Researchers Are Testing a New Stem Cell Therapy in the Hopes of Saving Millions from Blindness
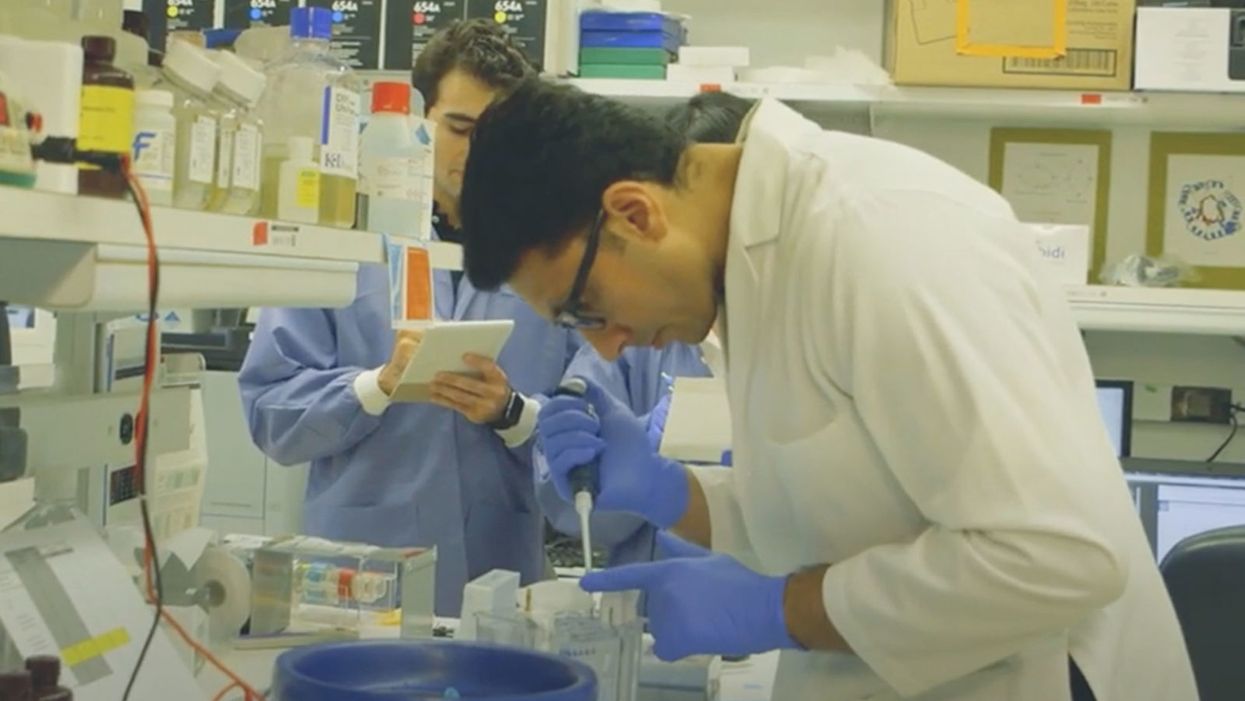
NIH researchers in Kapil Bharti's lab work toward the development of induced pluripotent stem cells to treat dry age-related macular degeneration.
Of all the infirmities of old age, failing sight is among the cruelest. It can mean the end not only of independence, but of a whole spectrum of joys—from gazing at a sunset or a grandchild's face to reading a novel or watching TV.
The Phase 1 trial will likely run through 2022, followed by a larger Phase 2 trial that could last another two or three years.
The leading cause of vision loss in people over 55 is age-related macular degeneration, or AMD, which afflicts an estimated 11 million Americans. As photoreceptors in the macula (the central part of the retina) die off, patients experience increasingly severe blurring, dimming, distortions, and blank spots in one or both eyes.
The disorder comes in two varieties, "wet" and "dry," both driven by a complex interaction of genetic, environmental, and lifestyle factors. It begins when deposits of cellular debris accumulate beneath the retinal pigment epithelium (RPE)—a layer of cells that nourish and remove waste products from the photoreceptors above them. In wet AMD, this process triggers the growth of abnormal, leaky blood vessels that damage the photoreceptors. In dry AMD, which accounts for 80 to 90 percent of cases, RPE cells atrophy, causing photoreceptors to wither away. Wet AMD can be controlled in about a quarter of patients, usually by injections of medication into the eye. For dry AMD, no effective remedy exists.
Stem Cells: Promise and Perils
Over the past decade, stem cell therapy has been widely touted as a potential treatment for AMD. The idea is to augment a patient's ailing RPE cells with healthy ones grown in the lab. A few small clinical trials have shown promising results. In a study published in 2018, for example, a University of Southern California team cultivated RPE tissue from embryonic stem cells on a plastic matrix and transplanted it into the retinas of four patients with advanced dry AMD. Because the trial was designed to test safety rather than efficacy, lead researcher Amir Kashani told a reporter, "we didn't expect that replacing RPE cells would return a significant amount of vision." Yet acuity improved substantially in one recipient, and the others regained their lost ability to focus on an object.
Therapies based on embryonic stem cells, however, have two serious drawbacks: Using fetal cell lines raises ethical issues, and such treatments require the patient to take immunosuppressant drugs (which can cause health problems of their own) to prevent rejection. That's why some experts favor a different approach—one based on induced pluripotent stem cells (iPSCs). Such cells, first produced in 2006, are made by returning adult cells to an undifferentiated state, and then using chemicals to reprogram them as desired. Treatments grown from a patient's own tissues could sidestep both hurdles associated with embryonic cells.
At least hypothetically. Today, the only stem cell therapies approved by the U.S. Food and Drug Administration (FDA) are umbilical cord-derived products for various blood and immune disorders. Although scientists are probing the use of embryonic stem cells or iPSCs for conditions ranging from diabetes to Parkinson's disease, such applications remain experimental—or fraudulent, as a growing number of patients treated at unlicensed "stem cell clinics" have painfully learned. (Some have gone blind after receiving bogus AMD therapies at those facilities.)
Last December, researchers at the National Eye Institute in Bethesda, Maryland, began enrolling patients with dry AMD in the country's first clinical trial using tissue grown from the patients' own stem cells. Led by biologist Kapil Bharti, the team intends to implant custom-made RPE cells in 12 recipients. If the effort pans out, it could someday save the sight of countless oldsters.
That, however, is what's technically referred to as a very big "if."
The First Steps
Bharti's trial is not the first in the world to use patient-derived iPSCs to treat age-related macular degeneration. In 2013, Japanese researchers implanted such cells into the eyes of a 77-year-old woman with wet AMD; after a year, her vision had stabilized, and she no longer needed injections to keep abnormal blood vessels from forming. A second patient was scheduled for surgery—but the procedure was canceled after the lab-grown RPE cells showed signs of worrisome mutations. That incident illustrates one potential problem with using stem cells: Under some circumstances, the cells or the tissue they form could turn cancerous.
"The knowledge and expertise we're gaining can be applied to many other iPSC-based therapies."
Bharti and his colleagues have gone to great lengths to avoid such outcomes. "Our process is significantly different," he told me in a phone interview. His team begins with patients' blood stem cells, which appear to be more genomically stable than the skin cells that the Japanese group used. After converting the blood cells to RPE stem cells, his team cultures them in a single layer on a biodegradable scaffold, which helps them grow in an orderly manner. "We think this material gives us a big advantage," Bharti says. The team uses a machine-learning algorithm to identify optimal cell structure and ensure quality control.
It takes about six months for a patch of iPSCs to become viable RPE cells. When they're ready, a surgeon uses a specially-designed tool to insert the tiny structure into the retina. Within days, the scaffold melts away, enabling the transplanted RPE cells to integrate fully into their new environment. Bharti's team initially tested their method on rats and pigs with eye damage mimicking AMD. The study, published in January 2019 in Science Translational Medicine, found that at ten weeks, the implanted RPE cells continued to function normally and protected neighboring photoreceptors from further deterioration. No trace of mutagenesis appeared.
Encouraged by these results, Bharti began recruiting human subjects. The Phase 1 trial will likely run through 2022, followed by a larger Phase 2 trial that could last another two or three years. FDA approval would require an even larger Phase 3 trial, with a decision expected sometime between 2025 and 2028—that is, if nothing untoward happens before then. One unknown (among many) is whether implanted cells can thrive indefinitely under the biochemically hostile conditions of an eye with AMD.
"Most people don't have a sense of just how long it takes to get something like this to work, and how many failures—even disasters—there are along the way," says Marco Zarbin, professor and chair of Ophthalmology and visual science at Rutgers New Jersey Medical School and co-editor of the book Cell-Based Therapy for Degenerative Retinal Diseases. "The first kidney transplant was done in 1933. But the first successful kidney transplant was in 1954. That gives you a sense of the time frame. We're really taking the very first steps in this direction."
Looking Ahead
Even if Bharti's method proves safe and effective, there's the question of its practicality. "My sense is that using induced pluripotent stem cells to treat the patient from whom they're derived is a very expensive undertaking," Zarbin observes. "So you'd have to have a very dramatic clinical benefit to justify that cost."
Bharti concedes that the price of iPSC therapy is likely to be high, given that each "dose" is formulated for a single individual, requires months to manufacture, and must be administered via microsurgery. Still, he expects economies of scale and production to emerge with time. "We're working on automating several steps of the process," he explains. "When that kicks in, a technician will be able to make products for 10 or 20 people at once, so the cost will drop proportionately."
Meanwhile, other researchers are pressing ahead with therapies for AMD using embryonic stem cells, which could be mass-produced to treat any patient who needs them. But should that approach eventually win FDA approval, Bharti believes there will still be room for a technique that requires neither fetal cell lines nor immunosuppression.
And not only for eye ailments. "The knowledge and expertise we're gaining can be applied to many other iPSC-based therapies," says the scientist, who is currently consulting with several companies that are developing such treatments. "I'm hopeful that we can leverage these approaches for a wide range of applications, whether it's for vision or across the body."
NEI launches iPS cell therapy trial for dry AMD
DNA- and RNA-based electronic implants may revolutionize healthcare
The test tubes contain tiny DNA/enzyme-based circuits, which comprise TRUMPET, a new type of electronic device, smaller than a cell.
Implantable electronic devices can significantly improve patients’ quality of life. A pacemaker can encourage the heart to beat more regularly. A neural implant, usually placed at the back of the skull, can help brain function and encourage higher neural activity. Current research on neural implants finds them helpful to patients with Parkinson’s disease, vision loss, hearing loss, and other nerve damage problems. Several of these implants, such as Elon Musk’s Neuralink, have already been approved by the FDA for human use.
Yet, pacemakers, neural implants, and other such electronic devices are not without problems. They require constant electricity, limited through batteries that need replacements. They also cause scarring. “The problem with doing this with electronics is that scar tissue forms,” explains Kate Adamala, an assistant professor of cell biology at the University of Minnesota Twin Cities. “Anytime you have something hard interacting with something soft [like muscle, skin, or tissue], the soft thing will scar. That's why there are no long-term neural implants right now.” To overcome these challenges, scientists are turning to biocomputing processes that use organic materials like DNA and RNA. Other promised benefits include “diagnostics and possibly therapeutic action, operating as nanorobots in living organisms,” writes Evgeny Katz, a professor of bioelectronics at Clarkson University, in his book DNA- And RNA-Based Computing Systems.
While a computer gives these inputs in binary code or "bits," such as a 0 or 1, biocomputing uses DNA strands as inputs, whether double or single-stranded, and often uses fluorescent RNA as an output.
Adamala’s research focuses on developing such biocomputing systems using DNA, RNA, proteins, and lipids. Using these molecules in the biocomputing systems allows the latter to be biocompatible with the human body, resulting in a natural healing process. In a recent Nature Communications study, Adamala and her team created a new biocomputing platform called TRUMPET (Transcriptional RNA Universal Multi-Purpose GatE PlaTform) which acts like a DNA-powered computer chip. “These biological systems can heal if you design them correctly,” adds Adamala. “So you can imagine a computer that will eventually heal itself.”
The basics of biocomputing
Biocomputing and regular computing have many similarities. Like regular computing, biocomputing works by running information through a series of gates, usually logic gates. A logic gate works as a fork in the road for an electronic circuit. The input will travel one way or another, giving two different outputs. An example logic gate is the AND gate, which has two inputs (A and B) and two different results. If both A and B are 1, the AND gate output will be 1. If only A is 1 and B is 0, the output will be 0 and vice versa. If both A and B are 0, the result will be 0. While a computer gives these inputs in binary code or "bits," such as a 0 or 1, biocomputing uses DNA strands as inputs, whether double or single-stranded, and often uses fluorescent RNA as an output. In this case, the DNA enters the logic gate as a single or double strand.
If the DNA is double-stranded, the system “digests” the DNA or destroys it, which results in non-fluorescence or “0” output. Conversely, if the DNA is single-stranded, it won’t be digested and instead will be copied by several enzymes in the biocomputing system, resulting in fluorescent RNA or a “1” output. And the output for this type of binary system can be expanded beyond fluorescence or not. For example, a “1” output might be the production of the enzyme insulin, while a “0” may be that no insulin is produced. “This kind of synergy between biology and computation is the essence of biocomputing,” says Stephanie Forrest, a professor and the director of the Biodesign Center for Biocomputing, Security and Society at Arizona State University.
Biocomputing circles are made of DNA, RNA, proteins and even bacteria.
Evgeny Katz
The TRUMPET’s promise
Depending on whether the biocomputing system is placed directly inside a cell within the human body, or run in a test-tube, different environmental factors play a role. When an output is produced inside a cell, the cell's natural processes can amplify this output (for example, a specific protein or DNA strand), creating a solid signal. However, these cells can also be very leaky. “You want the cells to do the thing you ask them to do before they finish whatever their businesses, which is to grow, replicate, metabolize,” Adamala explains. “However, often the gate may be triggered without the right inputs, creating a false positive signal. So that's why natural logic gates are often leaky." While biocomputing outside a cell in a test tube can allow for tighter control over the logic gates, the outputs or signals cannot be amplified by a cell and are less potent.
TRUMPET, which is smaller than a cell, taps into both cellular and non-cellular biocomputing benefits. “At its core, it is a nonliving logic gate system,” Adamala states, “It's a DNA-based logic gate system. But because we use enzymes, and the readout is enzymatic [where an enzyme replicates the fluorescent RNA], we end up with signal amplification." This readout means that the output from the TRUMPET system, a fluorescent RNA strand, can be replicated by nearby enzymes in the platform, making the light signal stronger. "So it combines the best of both worlds,” Adamala adds.
These organic-based systems could detect cancer cells or low insulin levels inside a patient’s body.
The TRUMPET biocomputing process is relatively straightforward. “If the DNA [input] shows up as single-stranded, it will not be digested [by the logic gate], and you get this nice fluorescent output as the RNA is made from the single-stranded DNA, and that's a 1,” Adamala explains. "And if the DNA input is double-stranded, it gets digested by the enzymes in the logic gate, and there is no RNA created from the DNA, so there is no fluorescence, and the output is 0." On the story's leading image above, if the tube is "lit" with a purple color, that is a binary 1 signal for computing. If it's "off" it is a 0.
While still in research, TRUMPET and other biocomputing systems promise significant benefits to personalized healthcare and medicine. These organic-based systems could detect cancer cells or low insulin levels inside a patient’s body. The study’s lead author and graduate student Judee Sharon is already beginning to research TRUMPET's ability for earlier cancer diagnoses. Because the inputs for TRUMPET are single or double-stranded DNA, any mutated or cancerous DNA could theoretically be detected from the platform through the biocomputing process. Theoretically, devices like TRUMPET could be used to detect cancer and other diseases earlier.
Adamala sees TRUMPET not only as a detection system but also as a potential cancer drug delivery system. “Ideally, you would like the drug only to turn on when it senses the presence of a cancer cell. And that's how we use the logic gates, which work in response to inputs like cancerous DNA. Then the output can be the production of a small molecule or the release of a small molecule that can then go and kill what needs killing, in this case, a cancer cell. So we would like to develop applications that use this technology to control the logic gate response of a drug’s delivery to a cell.”
Although platforms like TRUMPET are making progress, a lot more work must be done before they can be used commercially. “The process of translating mechanisms and architecture from biology to computing and vice versa is still an art rather than a science,” says Forrest. “It requires deep computer science and biology knowledge,” she adds. “Some people have compared interdisciplinary science to fusion restaurants—not all combinations are successful, but when they are, the results are remarkable.”
Crickets are low on fat, high on protein, and can be farmed sustainably. They are also crunchy.
In today’s podcast episode, Leaps.org Deputy Editor Lina Zeldovich speaks about the health and ecological benefits of farming crickets for human consumption with Bicky Nguyen, who joins Lina from Vietnam. Bicky and her business partner Nam Dang operate an insect farm named CricketOne. Motivated by the idea of sustainable and healthy protein production, they started their unconventional endeavor a few years ago, despite numerous naysayers who didn’t believe that humans would ever consider munching on bugs.
Yet, making creepy crawlers part of our diet offers many health and planetary advantages. Food production needs to match the rise in global population, estimated to reach 10 billion by 2050. One challenge is that some of our current practices are inefficient, polluting and wasteful. According to nonprofit EarthSave.org, it takes 2,500 gallons of water, 12 pounds of grain, 35 pounds of topsoil and the energy equivalent of one gallon of gasoline to produce one pound of feedlot beef, although exact statistics vary between sources.
Meanwhile, insects are easy to grow, high on protein and low on fat. When roasted with salt, they make crunchy snacks. When chopped up, they transform into delicious pâtes, says Bicky, who invents her own cricket recipes and serves them at industry and public events. Maybe that’s why some research predicts that edible insects market may grow to almost $10 billion by 2030. Tune in for a delectable chat on this alternative and sustainable protein.
Listen on Apple | Listen on Spotify | Listen on Stitcher | Listen on Amazon | Listen on Google
Further reading:
More info on Bicky Nguyen
https://yseali.fulbright.edu.vn/en/faculty/bicky-n...
The environmental footprint of beef production
https://www.earthsave.org/environment.htm
https://www.watercalculator.org/news/articles/beef-king-big-water-footprints/
https://www.frontiersin.org/articles/10.3389/fsufs.2019.00005/full
https://ourworldindata.org/carbon-footprint-food-methane
Insect farming as a source of sustainable protein
https://www.insectgourmet.com/insect-farming-growing-bugs-for-protein/
https://www.sciencedirect.com/topics/agricultural-and-biological-sciences/insect-farming
Cricket flour is taking the world by storm
https://www.cricketflours.com/
https://talk-commerce.com/blog/what-brands-use-cricket-flour-and-why/
Lina Zeldovich has written about science, medicine and technology for Popular Science, Smithsonian, National Geographic, Scientific American, Reader’s Digest, the New York Times and other major national and international publications. A Columbia J-School alumna, she has won several awards for her stories, including the ASJA Crisis Coverage Award for Covid reporting, and has been a contributing editor at Nautilus Magazine. In 2021, Zeldovich released her first book, The Other Dark Matter, published by the University of Chicago Press, about the science and business of turning waste into wealth and health. You can find her on http://linazeldovich.com/ and @linazeldovich.