“Young Blood” Transfusions Are Not Ready For Primetime – Yet
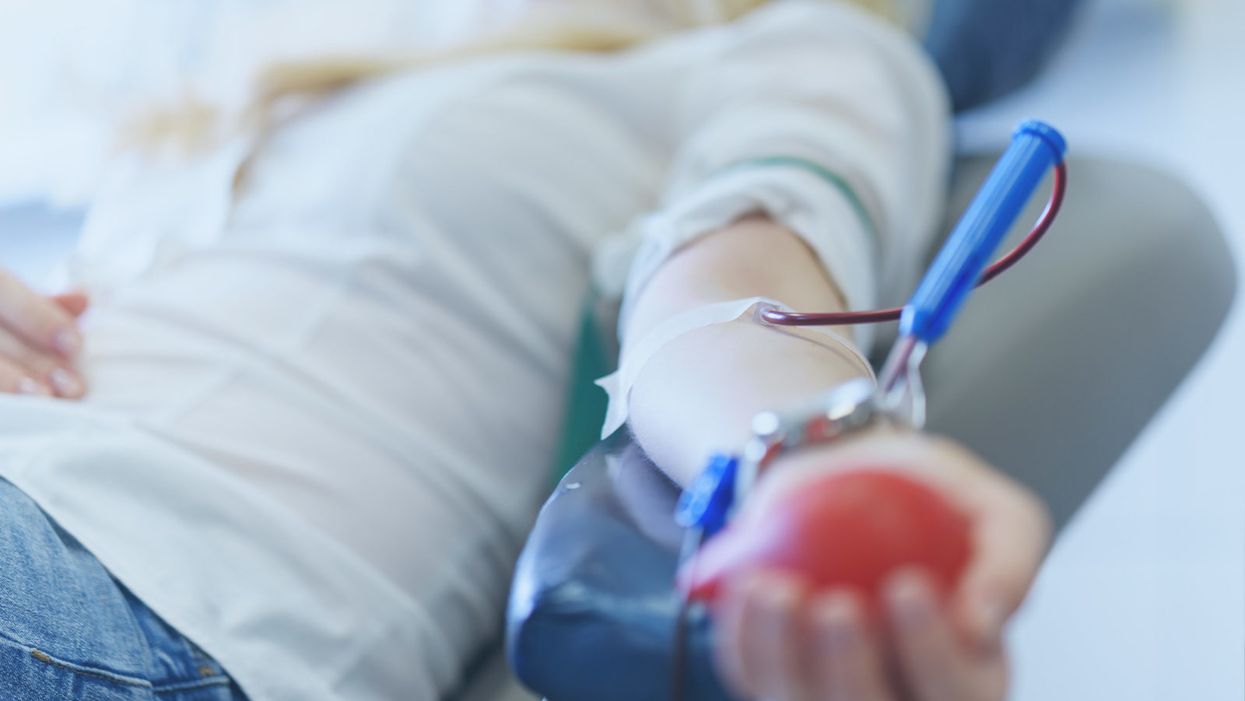
A young woman donates blood.
The world of dementia research erupted into cheers when news of the first real victory in a clinical trial against Alzheimer's Disease in over a decade was revealed last October.
By connecting the circulatory systems of a young and an old mouse, the regenerative potential of the young mouse decreased, and the old mouse became healthier.
Alzheimer's treatments have been famously difficult to develop; 99 percent of the 200-plus such clinical trials since 2000 have utterly failed. Even the few slight successes have failed to produce what is called 'disease modifying' agents that really help people with the disease. This makes the success, by the midsize Spanish pharma company Grifols, worthy of special attention.
However, the specifics of the Grifols treatment, a process called plasmapheresis, are atypical for another reason - they did not give patients a small molecule or an elaborate gene therapy, but rather simply the most common component of normal human blood plasma, a protein called albumin. A large portion of the patients' normal plasma was removed, and then a sterile solution of albumin was infused back into them to keep their overall blood volume relatively constant.
So why does replacing Alzheimer's patients' plasma with albumin seem to help their brains? One theory is that the action is direct. Alzheimer's patients have low levels of serum albumin, which is needed to clear out the plaques of amyloid that slowly build up in the brain. Supplementing those patients with extra albumin boosts their ability to clear the plaques and improves brain health. However, there is also evidence suggesting that the problem may be something present in the plasma of the sick person and pulling their plasma out and replacing it with a filler, like an albumin solution, may be what creates the purported benefit.
This scientific question is the tip of an iceberg that goes far beyond Alzheimer's Disease and albumin, to a debate that has been waged on the pages of scientific journals about the secrets of using young, healthy blood to extend youth and health.
This debate started long before the Grifols data was released, in 2014 when a group of researchers at Stanford found that by connecting the circulatory systems of a young and an old mouse, the regenerative potential of the young mouse decreased, and the old mouse became healthier. There was something either present in young blood that allowed tissues to regenerate, or something present in old blood that prevented regeneration. Whatever the biological reason, the effects in the experiment were extraordinary, providing a startling boost in health in the older mouse.
After the initial findings, multiple research groups got to work trying to identify the "active factor" of regeneration (or the inhibitor of that regeneration). They soon uncovered a variety of compounds such as insulin-like growth factor 1 (IGF1), CCL11, and GDF11, but none seemed to provide all the answers researchers were hoping for, with a number of high-profile retractions based on unsound experimental practices, or inconclusive data.
Years of research later, the simplest conclusion is that the story of plasma regeneration is not simple - there isn't a switch in our blood we can flip to turn back our biological clocks. That said, these hypotheses are far from dead, and many researchers continue to explore the possibility of using the rejuvenating ability of youthful plasma to treat a variety of diseases of aging.
But the bold claims of improved vigor thanks to young blood are so far unsupported by clinical evidence.
The data remain intriguing because of the astounding results from the conjoined circulatory system experiments. The current surge in interest in studying the biology of aging is likely to produce a new crop of interesting results in the next few years. Both CCL11 and GDF11 are being researched as potential drug targets by two startups, Alkahest and Elevian, respectively.
Without clarity on a single active factor driving rejuvenation, it's tempting to try a simpler approach: taking actual blood plasma provided by young people and infusing it into elderly subjects. This is what at least one startup company, Ambrosia, is now offering in five commercial clinics across the U.S. -- for $8,000 a liter.
By using whole plasma, the idea is to sidestep our ignorance, reaping the benefits of young plasma transfusion without knowing exactly what the active factors are that make the treatment work in mice. This space has attracted both established players in the plasmapheresis field – Alkahest and Grifols have teamed up to test fractions of whole plasma in Alzheimer's and Parkinson's – but also direct-to-consumer operations like Ambrosia that just want to offer patients access to treatments without regulatory oversight.
But the bold claims of improved vigor thanks to young blood are so far unsupported by clinical evidence. We simply haven't performed trials to test whether dosing a mostly healthy person with plasma can slow down aging, at least not yet. There is some evidence that plasma replacement works in mice, yes, but those experiments are all done in very different systems than what a human receiving young plasma might experience. To date, I have not seen any plasma transfusion clinic doing young blood plasmapheresis propose a clinical trial that is anything more than a shallow advertisement for their procedures.
The efforts I have seen to perform prophylactic plasmapheresis will fail to impact societal health. Without clearly defined endpoints and proper clinical trials, we won't know whether the procedure really lowers the risk of disease or helps with conditions of aging. So even if their hypothesis is correct, the lack of strong evidence to fall back on means that the procedure will never spread beyond the fringe groups willing to take the risk. If their hypothesis is wrong, then people are paying a huge amount of money for false hope, just as they do, sadly, at the phony stem cell clinics that started popping up all through the 2000s when stem cell hype was at its peak.
Until then, prophylactic plasma transfusions will be the domain of the optimistic and the gullible.
The real progress in the field will be made slowly, using carefully defined products either directly isolated from blood or targeting a bloodborne factor, just as the serious pharma and biotech players are doing already.
The field will progress in stages, first creating and carefully testing treatments for well-defined diseases, and only then will it progress to large-scale clinical trials in relatively healthy people to look for the prevention of disease. Most of us will choose to wait for this second stage of trials before undergoing any new treatments. Until then, prophylactic plasma transfusions will be the domain of the optimistic and the gullible.
Nobel Prize goes to technology for mRNA vaccines
Katalin Karikó, pictured, and Drew Weissman won the Nobel Prize for advances in mRNA research that led to the first Covid vaccines.
When Drew Weissman received a call from Katalin Karikó in the early morning hours this past Monday, he assumed his longtime research partner was calling to share a nascent, nagging idea. Weissman, a professor of medicine at the Perelman School of Medicine at the University of Pennsylvania, and Karikó, a professor at Szeged University and an adjunct professor at UPenn, both struggle with sleep disturbances. Thus, middle-of-the-night discourses between the two, often over email, has been a staple of their friendship. But this time, Karikó had something more pressing and exciting to share: They had won the 2023 Nobel Prize in Physiology or Medicine.
The work for which they garnered the illustrious award and its accompanying $1,000,000 cash windfall was completed about two decades ago, wrought through long hours in the lab over many arduous years. But humanity collectively benefited from its life-saving outcome three years ago, when both Moderna and Pfizer/BioNTech’s mRNA vaccines against COVID were found to be safe and highly effective at preventing severe disease. Billions of doses have since been given out to protect humans from the upstart viral scourge.
“I thought of going somewhere else, or doing something else,” said Katalin Karikó. “I also thought maybe I’m not good enough, not smart enough. I tried to imagine: Everything is here, and I just have to do better experiments.”
Unlocking the power of mRNA
Weissman and Karikó unlocked mRNA vaccines for the world back in the early 2000s when they made a key breakthrough. Messenger RNA molecules are essentially instructions for cells’ ribosomes to make specific proteins, so in the 1980s and 1990s, researchers started wondering if sneaking mRNA into the body could trigger cells to manufacture antibodies, enzymes, or growth agents for protecting against infection, treating disease, or repairing tissues. But there was a big problem: injecting this synthetic mRNA triggered a dangerous, inflammatory immune response resulting in the mRNA’s destruction.
While most other researchers chose not to tackle this perplexing problem to instead pursue more lucrative and publishable exploits, Karikó stuck with it. The choice sent her academic career into depressing doldrums. Nobody would fund her work, publications dried up, and after six years as an assistant professor at the University of Pennsylvania, Karikó got demoted. She was going backward.
“I thought of going somewhere else, or doing something else,” Karikó told Stat in 2020. “I also thought maybe I’m not good enough, not smart enough. I tried to imagine: Everything is here, and I just have to do better experiments.”
A tale of tenacity
Collaborating with Drew Weissman, a new professor at the University of Pennsylvania, in the late 1990s helped provide Karikó with the tenacity to continue. Weissman nurtured a goal of developing a vaccine against HIV-1, and saw mRNA as a potential way to do it.
“For the 20 years that we’ve worked together before anybody knew what RNA is, or cared, it was the two of us literally side by side at a bench working together,” Weissman said in an interview with Adam Smith of the Nobel Foundation.
In 2005, the duo made their 2023 Nobel Prize-winning breakthrough, detailing it in a relatively small journal, Immunity. (Their paper was rejected by larger journals, including Science and Nature.) They figured out that chemically modifying the nucleoside bases that make up mRNA allowed the molecule to slip past the body’s immune defenses. Karikó and Weissman followed up that finding by creating mRNA that’s more efficiently translated within cells, greatly boosting protein production. In 2020, scientists at Moderna and BioNTech (where Karikó worked from 2013 to 2022) rushed to craft vaccines against COVID, putting their methods to life-saving use.
The future of vaccines
Buoyed by the resounding success of mRNA vaccines, scientists are now hurriedly researching ways to use mRNA medicine against other infectious diseases, cancer, and genetic disorders. The now ubiquitous efforts stand in stark contrast to Karikó and Weissman’s previously unheralded struggles years ago as they doggedly worked to realize a shared dream that so many others shied away from. Katalin Karikó and Drew Weissman were brave enough to walk a scientific path that very well could have ended in a dead end, and for that, they absolutely deserve their 2023 Nobel Prize.
This article originally appeared on Big Think, home of the brightest minds and biggest ideas of all time.
Scientists turn pee into power in Uganda
With conventional fuel cells as their model, researchers learned to use similar chemical reactions to make a fuel from microbes in pee.
At the edge of a dirt road flanked by trees and green mountains outside the town of Kisoro, Uganda, sits the concrete building that houses Sesame Girls School, where girls aged 11 to 19 can live, learn and, at least for a while, safely use a toilet. In many developing regions, toileting at night is especially dangerous for children. Without electrical power for lighting, kids may fall into the deep pits of the latrines through broken or unsteady floorboards. Girls are sometimes assaulted by men who hide in the dark.
For the Sesame School girls, though, bright LED lights, connected to tiny gadgets, chased the fears away. They got to use new, clean toilets lit by the power of their own pee. Some girls even used the light provided by the latrines to study.
Urine, whether animal or human, is more than waste. It’s a cheap and abundant resource. Each day across the globe, 8.1 billion humans make 4 billion gallons of pee. Cows, pigs, deer, elephants and other animals add more. By spending money to get rid of it, we waste a renewable resource that can serve more than one purpose. Microorganisms that feed on nutrients in urine can be used in a microbial fuel cell that generates electricity – or "pee power," as the Sesame girls called it.
Plus, urine contains water, phosphorus, potassium and nitrogen, the key ingredients plants need to grow and survive. Human urine could replace about 25 percent of current nitrogen and phosphorous fertilizers worldwide and could save water for gardens and crops. The average U.S. resident flushes a toilet bowl containing only pee and paper about six to seven times a day, which adds up to about 3,500 gallons of water down per year. Plus cows in the U.S. produce 231 gallons of the stuff each year.
Pee power
A conventional fuel cell uses chemical reactions to produce energy, as electrons move from one electrode to another to power a lightbulb or phone. Ioannis Ieropoulos, a professor and chair of Environmental Engineering at the University of Southampton in England, realized the same type of reaction could be used to make a fuel from microbes in pee.
Bacterial species like Shewanella oneidensis and Pseudomonas aeruginosa can consume carbon and other nutrients in urine and pop out electrons as a result of their digestion. In a microbial fuel cell, one electrode is covered in microbes, immersed in urine and kept away from oxygen. Another electrode is in contact with oxygen. When the microbes feed on nutrients, they produce the electrons that flow through the circuit from one electrod to another to combine with oxygen on the other side. As long as the microbes have fresh pee to chomp on, electrons keep flowing. And after the microbes are done with the pee, it can be used as fertilizer.
These microbes are easily found in wastewater treatment plants, ponds, lakes, rivers or soil. Keeping them alive is the easy part, says Ieropoulos. Once the cells start producing stable power, his group sequences the microbes and keeps using them.
Like many promising technologies, scaling these devices for mass consumption won’t be easy, says Kevin Orner, a civil engineering professor at West Virginia University. But it’s moving in the right direction. Ieropoulos’s device has shrunk from the size of about three packs of cards to a large glue stick. It looks and works much like a AAA battery and produce about the same power. By itself, the device can barely power a light bulb, but when stacked together, they can do much more—just like photovoltaic cells in solar panels. His lab has produced 1760 fuel cells stacked together, and with manufacturing support, there’s no theoretical ceiling, he says.
Although pure urine produces the most power, Ieropoulos’s devices also work with the mixed liquids of the wastewater treatment plants, so they can be retrofit into urban wastewater utilities.
This image shows how the pee-powered system works. Pee feeds bacteria in the stack of fuel cells (1), which give off electrons (2) stored in parallel cylindrical cells (3). These cells are connected to a voltage regulator (4), which smooths out the electrical signal to ensure consistent power to the LED strips lighting the toilet.
Courtesy Ioannis Ieropoulos
Key to the long-term success of any urine reclamation effort, says Orner, is avoiding what he calls “parachute engineering”—when well-meaning scientists solve a problem with novel tech and then abandon it. “The way around that is to have either the need come from the community or to have an organization in a community that is committed to seeing a project operate and maintained,” he says.
Success with urine reclamation also depends on the economy. “If energy prices are low, it may not make sense to recover energy,” says Orner. “But right now, fertilizer prices worldwide are generally pretty high, so it may make sense to recover fertilizer and nutrients.” There are obstacles, too, such as few incentives for builders to incorporate urine recycling into new construction. And any hiccups like leaks or waste seepage will cost builders money and reputation. Right now, Orner says, the risks are just too high.
Despite the challenges, Ieropoulos envisions a future in which urine is passed through microbial fuel cells at wastewater treatment plants, retrofitted septic tanks, and building basements, and is then delivered to businesses to use as agricultural fertilizers. Although pure urine produces the most power, Ieropoulos’s devices also work with the mixed liquids of the wastewater treatment plants, so they can be retrofitted into urban wastewater utilities where they can make electricity from the effluent. And unlike solar cells, which are a common target of theft in some areas, nobody wants to steal a bunch of pee.
When Ieropoulos’s team returned to wrap up their pilot project 18 months later, the school’s director begged them to leave the fuel cells in place—because they made a major difference in students’ lives. “We replaced it with a substantial photovoltaic panel,” says Ieropoulos, They couldn’t leave the units forever, he explained, because of intellectual property reasons—their funders worried about theft of both the technology and the idea. But the photovoltaic replacement could be stolen, too, leaving the girls in the dark.
The story repeated itself at another school, in Nairobi, Kenya, as well as in an informal settlement in Durban, South Africa. Each time, Ieropoulos vowed to return. Though the pandemic has delayed his promise, he is resolute about continuing his work—it is a moral and legal obligation. “We've made a commitment to ourselves and to the pupils,” he says. “That's why we need to go back.”
Urine as fertilizer
Modern day industrial systems perpetuate the broken cycle of nutrients. When plants grow, they use up nutrients the soil. We eat the plans and excrete some of the nutrients we pass them into rivers and oceans. As a result, farmers must keep fertilizing the fields while our waste keeps fertilizing the waterways, where the algae, overfertilized with nitrogen, phosphorous and other nutrients grows out of control, sucking up oxygen that other marine species need to live. Few global communities remain untouched by the related challenges this broken chain create: insufficient clean water, food, and energy, and too much human and animal waste.
The Rich Earth Institute in Vermont runs a community-wide urine nutrient recovery program, which collects urine from homes and businesses, transports it for processing, and then supplies it as fertilizer to local farms.
One solution to this broken cycle is reclaiming urine and returning it back to the land. The Rich Earth Institute in Vermont is one of several organizations around the world working to divert and save urine for agricultural use. “The urine produced by an adult in one day contains enough fertilizer to grow all the wheat in one loaf of bread,” states their website.
Notably, while urine is not entirely sterile, it tends to harbor fewer pathogens than feces. That’s largely because urine has less organic matter and therefore less food for pathogens to feed on, but also because the urinary tract and the bladder have built-in antimicrobial defenses that kill many germs. In fact, the Rich Earth Institute says it’s safe to put your own urine onto crops grown for home consumption. Nonetheless, you’ll want to dilute it first because pee usually has too much nitrogen and can cause “fertilizer burn” if applied straight without dilution. Other projects to turn urine into fertilizer are in progress in Niger, South Africa, Kenya, Ethiopia, Sweden, Switzerland, The Netherlands, Australia, and France.
Eleven years ago, the Institute started a program that collects urine from homes and businesses, transports it for processing, and then supplies it as fertilizer to local farms. By 2021, the program included 180 donors producing over 12,000 gallons of urine each year. This urine is helping to fertilize hay fields at four partnering farms. Orner, the West Virginia professor, sees it as a success story. “They've shown how you can do this right--implementing it at a community level scale."