Is Red Tape Depriving Patients of Life-Altering Therapies?
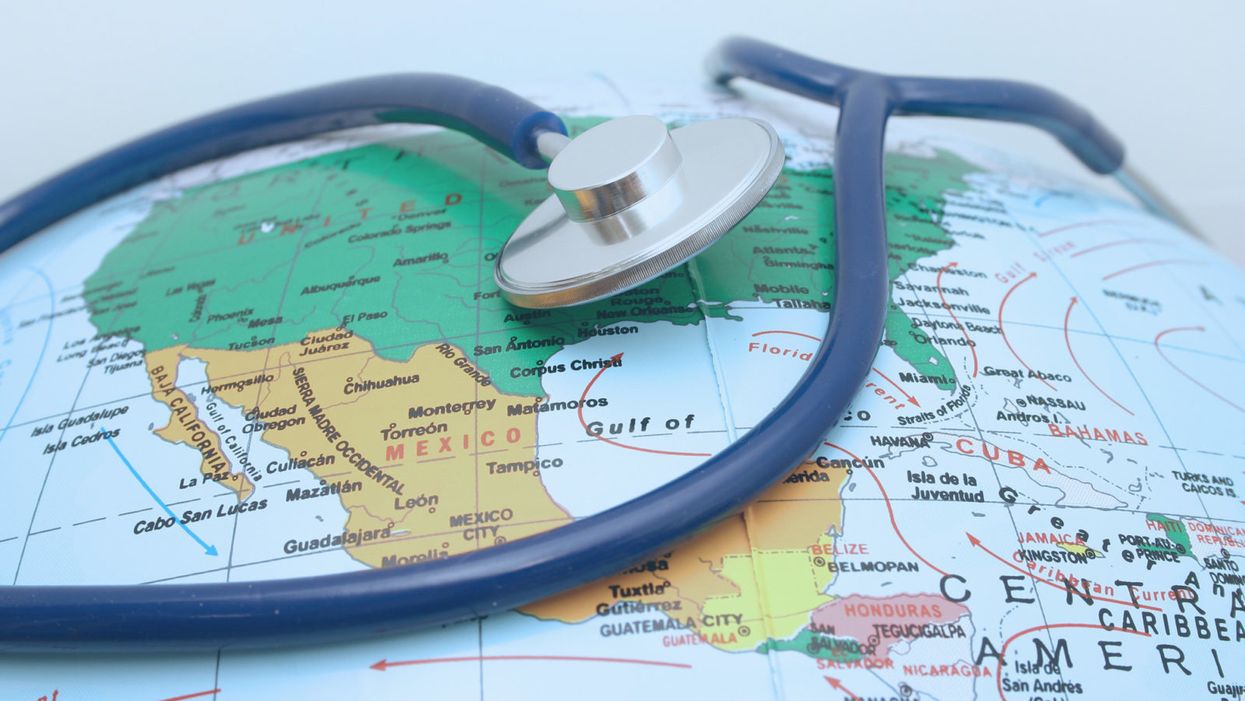
Medical treatment represented on a world map.
Rich Mancuso suffered from herpes for most of his adult life. The 49-year-old New Jersey resident was miserable. He had at least two to three outbreaks every month with painful and unsightly sores on his face and in his eyes, yet the drugs he took to control the disease had terrible side effects--agonizing headaches and severe stomach disturbances.
Last week, the FDA launched a criminal investigation to determine whether the biotech behind the vaccine had violated regulations.
So in 2016, he took an unusual step: he was flown to St. Kitt's, an island in the West Indies, where he participated in a clinical trial of a herpes vaccine, and received three injections of the experimental therapeutic during separate visits to the island. Within a year, his outbreaks stopped. "Nothing else worked," says Mancuso, who feels like he's gotten his life back. "And I've tried everything on the planet."
Mancuso was one of twenty genital herpes sufferers who were given the experimental vaccine in tests conducted on the Caribbean island and in hotel rooms near the campus of Southern Illinois University in Springfield where the vaccine's developer, microbiologist William Halford, was on the faculty. But these tests were conducted under the radar, without the approval or safety oversight of the Food and Drug Administration or an institutional review board (IRB), which routinely monitor human clinical trials of experimental drugs to make sure participants are protected.
Last week, the FDA launched a criminal investigation to determine whether anyone from SIU or Rational Vaccines, the biotech behind the vaccine, had violated regulations by aiding Halford's research. The SIU scientist was a microbiologist, not a medical doctor, which means that volunteers were not only injected with an unsanctioned experimental treatment but there wasn't even routine medical oversight.
On one side are scientists and government regulators with legitimate safety concerns....On the other are desperate patients and a dying scientist willing to go rogue in a foreign country.
Halford, who was stricken with a rare form of a nasal cancer, reportedly bypassed regulatory rules because the clock was ticking and he wanted to speed this potentially life-altering therapeutic to patients. "There was no way he had enough time to raise $100 million to test the drugs in the U.S.," says Mancuso, who became friends with Halford before he died in June of 2017 at age 48. "He knew if he didn't do something, his work would just die and no one would benefit. This was the only way."
But was it the only way? Once the truth about the trial came to light, public health officials in St. Kitt's disavowed the trial, saying they had not been notified that it was happening, and Southern Illinois University's medical school launched an investigation that ultimately led to the resignation of three employees, including a faculty member, a graduate student and Halford's widow. Investors in Rational Vaccines, including maverick Silicon Valley billionaire Peter Thiel, demanded that all FDA rules must be followed in future tests.
"Trials have to yield data that can be submitted to the FDA, which means certain requirements have to be met," says Jeffrey Kahn, a bioethicist at Johns Hopkins University in Baltimore. "These were renegade researchers who exposed people to unnecessary risks, which was hugely irresponsible. I don't know what they expected to do with the research. It was a waste of money and generated data that can't be used because no regulator would accept it."
But this story illuminates both sides of a thorny issue. On one side are scientists and government regulators with legitimate safety concerns who want to protect volunteers from very real risks—people have died even in closely monitored clinical trials. On the other, are desperate patients and a dying scientist willing to go rogue in a foreign country where there is far less regulatory scrutiny. "It's a balancing act," says Jennifer Miller, a medical ethicist at New York University and president of Bioethics International. "You really need to protect participants but you also want access to safe therapies."
"Safety is important, but being too cautious kills people, too—allowing them to just die without intervention seems to be the biggest harm."
This requirement—that tests show a drug is safe and effective before it can win regulatory approval--dates back to 1962, when the sedative thalidomide was shown to have caused thousands of birth defects in Europe. But clinical trials can be costly and often proceed at a glacial pace. Typically, companies shell out more than $2.5 billion over the course of the decade it normally takes to shepherd a new treatment through the three phases of testing before it wins FDA approval, according to a 2014 study by the Tufts Center for the Study of Drug Development. Yet only 11.8 percent of experimental therapies entering clinical tests eventually cross the finish line.
The upshot is that millions can suffer and thousands of people may die awaiting approvals for life saving drugs, according to Elizabeth Parrish, the founder and CEO of BioViva, a Seattle-based biotech that aims to provide data collection platforms to scientists doing overseas tests. "Going offshore to places where it's legal to take a therapeutic can created expedited routes for patients to get therapies for which there is a high level of need," she says. "Safety is important, but being too cautious kills people, too—allowing them to just die without intervention seems to be the biggest harm."
Parrish herself was frustrated with the slow pace of gene therapy trials; scientists worried about the risks associated with fixing mutant DNA. To prove a point, she traveled to a clinic in Colombia in 2015 where she was injected with two gene therapies that aim to improve muscle function and lengthen telomeres, the caps on the end of chromosomes that are linked to aging and genetic diseases. Six months later, the therapy seemed to have worked—her muscle mass had increased and her telomeres had grown by 9 percent, the equivalent of turning back 20 years of aging, according to her own account. Yet the treatments are still unavailable here in the U.S.
In the past decade, Latin American countries like Columbia, and Mexico in particular, have become an increasingly attractive test destination for multi-national drug companies and biotechs because of less red tape.
In the past decade, Latin American countries like Columbia, and Mexico in particular, have become an increasingly attractive test destination for multi-national drug companies and biotechs because of less red tape around testing emerging new science, like gene therapies or stem cells. Plus, clinical trials are cheaper to conduct, it's easier to recruit volunteers, especially ones who are treatment naïve, and these human tests can reveal whether local populations actually respond to a particular therapy. "We do have an exhaustive framework for running clinical trials that are aligned with international requirements," says Ernesto Albaga, an attorney with Hogan Lovells in Mexico City who specializes in the life sciences. "But our environment is still not as stringent as it is in other places, like the U.S."
The fact is American researchers are increasingly testing experimental drugs outside of the U.S., although virtually all of them are monitored by local scientists who serve as co-investigators. In 2017 alone, more than 86 percent of experimental drugs seeking FDA approval have been tested, at least in part, in foreign countries, like Mexico, China, Russia, Poland and South Africa, according to an analysis by STAT. However, in places without strict oversight, such as Russia and Georgia, results may be fraudulent, according to one 2017 report in the New England Journal of Medicine. And in developing countries, the poor can become guinea pigs. In the early 2000s, for example, a test in Uganda of an AIDS drug resulted in thousands of unreported serious adverse reactions and 14 deaths; in India, eight volunteers died during a test of the anti-clotting drug, Streptokinase—and test subjects didn't even know they were part of a clinical trials.
Still, "the world is changing," concludes Dr. Jennifer Miller of NYU. "We need to figure out how to get safe and effective drugs to patients more quickly without sacrificing too much protection."
DNA- and RNA-based electronic implants may revolutionize healthcare
The test tubes contain tiny DNA/enzyme-based circuits, which comprise TRUMPET, a new type of electronic device, smaller than a cell.
Implantable electronic devices can significantly improve patients’ quality of life. A pacemaker can encourage the heart to beat more regularly. A neural implant, usually placed at the back of the skull, can help brain function and encourage higher neural activity. Current research on neural implants finds them helpful to patients with Parkinson’s disease, vision loss, hearing loss, and other nerve damage problems. Several of these implants, such as Elon Musk’s Neuralink, have already been approved by the FDA for human use.
Yet, pacemakers, neural implants, and other such electronic devices are not without problems. They require constant electricity, limited through batteries that need replacements. They also cause scarring. “The problem with doing this with electronics is that scar tissue forms,” explains Kate Adamala, an assistant professor of cell biology at the University of Minnesota Twin Cities. “Anytime you have something hard interacting with something soft [like muscle, skin, or tissue], the soft thing will scar. That's why there are no long-term neural implants right now.” To overcome these challenges, scientists are turning to biocomputing processes that use organic materials like DNA and RNA. Other promised benefits include “diagnostics and possibly therapeutic action, operating as nanorobots in living organisms,” writes Evgeny Katz, a professor of bioelectronics at Clarkson University, in his book DNA- And RNA-Based Computing Systems.
While a computer gives these inputs in binary code or "bits," such as a 0 or 1, biocomputing uses DNA strands as inputs, whether double or single-stranded, and often uses fluorescent RNA as an output.
Adamala’s research focuses on developing such biocomputing systems using DNA, RNA, proteins, and lipids. Using these molecules in the biocomputing systems allows the latter to be biocompatible with the human body, resulting in a natural healing process. In a recent Nature Communications study, Adamala and her team created a new biocomputing platform called TRUMPET (Transcriptional RNA Universal Multi-Purpose GatE PlaTform) which acts like a DNA-powered computer chip. “These biological systems can heal if you design them correctly,” adds Adamala. “So you can imagine a computer that will eventually heal itself.”
The basics of biocomputing
Biocomputing and regular computing have many similarities. Like regular computing, biocomputing works by running information through a series of gates, usually logic gates. A logic gate works as a fork in the road for an electronic circuit. The input will travel one way or another, giving two different outputs. An example logic gate is the AND gate, which has two inputs (A and B) and two different results. If both A and B are 1, the AND gate output will be 1. If only A is 1 and B is 0, the output will be 0 and vice versa. If both A and B are 0, the result will be 0. While a computer gives these inputs in binary code or "bits," such as a 0 or 1, biocomputing uses DNA strands as inputs, whether double or single-stranded, and often uses fluorescent RNA as an output. In this case, the DNA enters the logic gate as a single or double strand.
If the DNA is double-stranded, the system “digests” the DNA or destroys it, which results in non-fluorescence or “0” output. Conversely, if the DNA is single-stranded, it won’t be digested and instead will be copied by several enzymes in the biocomputing system, resulting in fluorescent RNA or a “1” output. And the output for this type of binary system can be expanded beyond fluorescence or not. For example, a “1” output might be the production of the enzyme insulin, while a “0” may be that no insulin is produced. “This kind of synergy between biology and computation is the essence of biocomputing,” says Stephanie Forrest, a professor and the director of the Biodesign Center for Biocomputing, Security and Society at Arizona State University.
Biocomputing circles are made of DNA, RNA, proteins and even bacteria.
Evgeny Katz
The TRUMPET’s promise
Depending on whether the biocomputing system is placed directly inside a cell within the human body, or run in a test-tube, different environmental factors play a role. When an output is produced inside a cell, the cell's natural processes can amplify this output (for example, a specific protein or DNA strand), creating a solid signal. However, these cells can also be very leaky. “You want the cells to do the thing you ask them to do before they finish whatever their businesses, which is to grow, replicate, metabolize,” Adamala explains. “However, often the gate may be triggered without the right inputs, creating a false positive signal. So that's why natural logic gates are often leaky." While biocomputing outside a cell in a test tube can allow for tighter control over the logic gates, the outputs or signals cannot be amplified by a cell and are less potent.
TRUMPET, which is smaller than a cell, taps into both cellular and non-cellular biocomputing benefits. “At its core, it is a nonliving logic gate system,” Adamala states, “It's a DNA-based logic gate system. But because we use enzymes, and the readout is enzymatic [where an enzyme replicates the fluorescent RNA], we end up with signal amplification." This readout means that the output from the TRUMPET system, a fluorescent RNA strand, can be replicated by nearby enzymes in the platform, making the light signal stronger. "So it combines the best of both worlds,” Adamala adds.
These organic-based systems could detect cancer cells or low insulin levels inside a patient’s body.
The TRUMPET biocomputing process is relatively straightforward. “If the DNA [input] shows up as single-stranded, it will not be digested [by the logic gate], and you get this nice fluorescent output as the RNA is made from the single-stranded DNA, and that's a 1,” Adamala explains. "And if the DNA input is double-stranded, it gets digested by the enzymes in the logic gate, and there is no RNA created from the DNA, so there is no fluorescence, and the output is 0." On the story's leading image above, if the tube is "lit" with a purple color, that is a binary 1 signal for computing. If it's "off" it is a 0.
While still in research, TRUMPET and other biocomputing systems promise significant benefits to personalized healthcare and medicine. These organic-based systems could detect cancer cells or low insulin levels inside a patient’s body. The study’s lead author and graduate student Judee Sharon is already beginning to research TRUMPET's ability for earlier cancer diagnoses. Because the inputs for TRUMPET are single or double-stranded DNA, any mutated or cancerous DNA could theoretically be detected from the platform through the biocomputing process. Theoretically, devices like TRUMPET could be used to detect cancer and other diseases earlier.
Adamala sees TRUMPET not only as a detection system but also as a potential cancer drug delivery system. “Ideally, you would like the drug only to turn on when it senses the presence of a cancer cell. And that's how we use the logic gates, which work in response to inputs like cancerous DNA. Then the output can be the production of a small molecule or the release of a small molecule that can then go and kill what needs killing, in this case, a cancer cell. So we would like to develop applications that use this technology to control the logic gate response of a drug’s delivery to a cell.”
Although platforms like TRUMPET are making progress, a lot more work must be done before they can be used commercially. “The process of translating mechanisms and architecture from biology to computing and vice versa is still an art rather than a science,” says Forrest. “It requires deep computer science and biology knowledge,” she adds. “Some people have compared interdisciplinary science to fusion restaurants—not all combinations are successful, but when they are, the results are remarkable.”
Crickets are low on fat, high on protein, and can be farmed sustainably. They are also crunchy.
In today’s podcast episode, Leaps.org Deputy Editor Lina Zeldovich speaks about the health and ecological benefits of farming crickets for human consumption with Bicky Nguyen, who joins Lina from Vietnam. Bicky and her business partner Nam Dang operate an insect farm named CricketOne. Motivated by the idea of sustainable and healthy protein production, they started their unconventional endeavor a few years ago, despite numerous naysayers who didn’t believe that humans would ever consider munching on bugs.
Yet, making creepy crawlers part of our diet offers many health and planetary advantages. Food production needs to match the rise in global population, estimated to reach 10 billion by 2050. One challenge is that some of our current practices are inefficient, polluting and wasteful. According to nonprofit EarthSave.org, it takes 2,500 gallons of water, 12 pounds of grain, 35 pounds of topsoil and the energy equivalent of one gallon of gasoline to produce one pound of feedlot beef, although exact statistics vary between sources.
Meanwhile, insects are easy to grow, high on protein and low on fat. When roasted with salt, they make crunchy snacks. When chopped up, they transform into delicious pâtes, says Bicky, who invents her own cricket recipes and serves them at industry and public events. Maybe that’s why some research predicts that edible insects market may grow to almost $10 billion by 2030. Tune in for a delectable chat on this alternative and sustainable protein.
Listen on Apple | Listen on Spotify | Listen on Stitcher | Listen on Amazon | Listen on Google
Further reading:
More info on Bicky Nguyen
https://yseali.fulbright.edu.vn/en/faculty/bicky-n...
The environmental footprint of beef production
https://www.earthsave.org/environment.htm
https://www.watercalculator.org/news/articles/beef-king-big-water-footprints/
https://www.frontiersin.org/articles/10.3389/fsufs.2019.00005/full
https://ourworldindata.org/carbon-footprint-food-methane
Insect farming as a source of sustainable protein
https://www.insectgourmet.com/insect-farming-growing-bugs-for-protein/
https://www.sciencedirect.com/topics/agricultural-and-biological-sciences/insect-farming
Cricket flour is taking the world by storm
https://www.cricketflours.com/
https://talk-commerce.com/blog/what-brands-use-cricket-flour-and-why/
Lina Zeldovich has written about science, medicine and technology for Popular Science, Smithsonian, National Geographic, Scientific American, Reader’s Digest, the New York Times and other major national and international publications. A Columbia J-School alumna, she has won several awards for her stories, including the ASJA Crisis Coverage Award for Covid reporting, and has been a contributing editor at Nautilus Magazine. In 2021, Zeldovich released her first book, The Other Dark Matter, published by the University of Chicago Press, about the science and business of turning waste into wealth and health. You can find her on http://linazeldovich.com/ and @linazeldovich.