The Inside Story of Two Young Scientists Who Helped Make Moderna's Covid Vaccine Possible
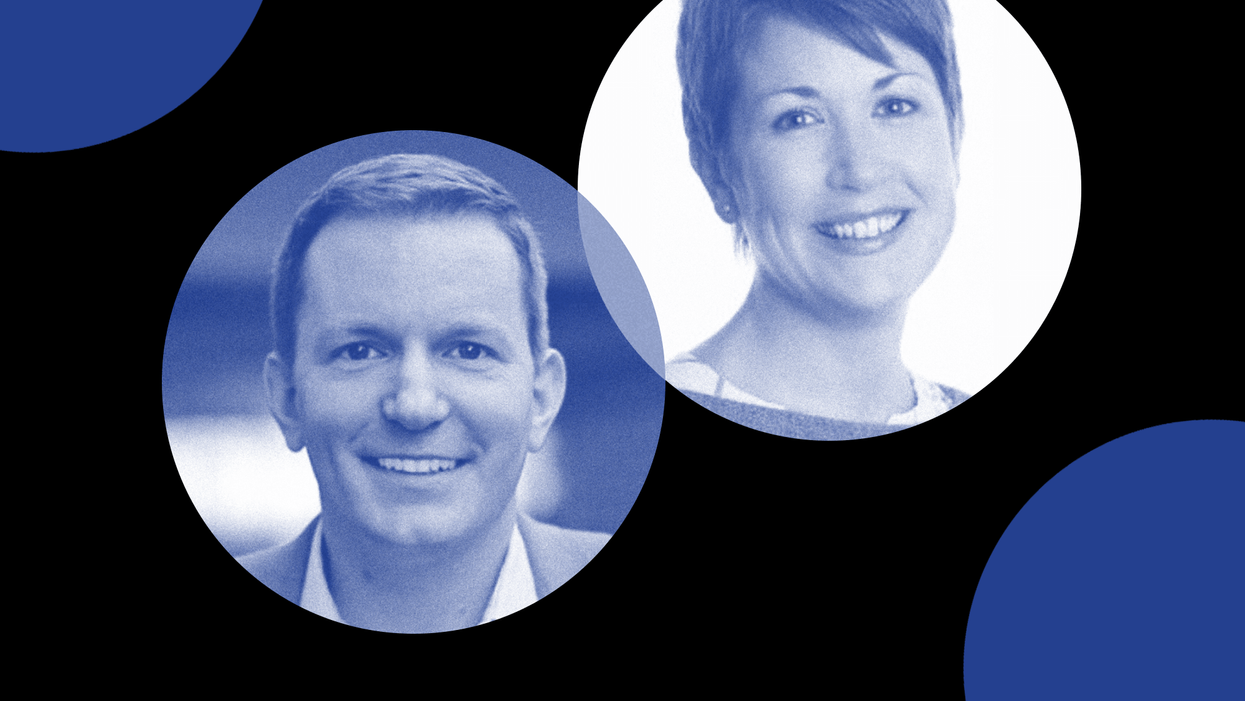
Scientists Jason Schrum and Kerry Benenato solved crucial challenges in mRNA vaccine development.
In early 2020, Moderna Inc. was a barely-known biotechnology company with an unproven approach. It wanted to produce messenger RNA molecules to carry instructions into the body, teaching it to ward off disease. Experts doubted the Boston-based company would meet success.
Today, Moderna is a pharmaceutical power thanks to its success developing an effective Covid-19 vaccine. The company is worth $124 billion, more than giants including GlaxoSmithKline and Sanofi, and evidence has emerged that Moderna's shots are more protective than those produced by Pfizer-BioNTech and other vaccine makers. Pressure is building on the company to deliver more of its doses to people around the world, especially in poorer countries, and Moderna is working on vaccines against other pathogens, including Zika, influenza and cytomegalovirus.
But Moderna encountered such difficulties over the course of its eleven-year history that some executives worried it wouldn't survive. Two unlikely scientists helped save the company. Their breakthroughs paved the way for Moderna's Covid-19 shots but their work has never been publicized nor have their contributions been properly appreciated.
Derrick Rossi, a scientist at MIT, and Noubar Afeyan, a Cambridge-based investor, launched Moderna in September 2010. Their idea was to create mRNA molecules capable of delivering instructions to the body's cells, directing them to make proteins to heal ailments and cure disease. Need a statin, immunosuppressive, or other drug or vaccine? Just use mRNA to send a message to the body's cells to produce it. Rossi and Afeyan were convinced injecting mRNA into the body could turn it into its own laboratory, generating specific medications or vaccines as needed.
At the time, the notion that one might be able to teach the body to make proteins bordered on heresy. Everyone knew mRNA was unstable and set off the body's immune system on its way into cells. But in the late 2000's, two scientists at the University of Pennsylvania, Katalin Karikó and Drew Weissman, had figured out how to modify mRNA's chemical building blocks so the molecule could escape the notice of the immune system and enter the cell. Rossi and Afeyan couldn't convince the University of Pennsylvania to license Karikó and Weissman's patent, however, stymying Moderna's early ambitions. At the same time, the Penn scientists' technique seemed more applicable to an academic lab than a biotech company that needed to produce drugs or shots consistently and in bulk. Rossi and Afeyan's new company needed their own solution to help mRNA evade the body's defenses.
Some of Moderna's founders doubted Schrum could find success and they worried if their venture was doomed from the start.
The Scientist Who Modified mRNA: Jason Schrum
In 2010, Afeyan's firm subleased laboratory space in the basement of another Cambridge biotech company to begin scientific work. Afeyan chose a young scientist on his staff, Jason Schrum, to be Moderna's first employee, charging him with getting mRNA into cells without relying on Karikó and Weissman's solutions.
Schrum seemed well suited for the task. Months earlier, he had received a PhD in biological chemistry at Harvard University, where he had focused on nucleotide chemistry. Schrum even had the look of someone who might do big things. The baby-faced twenty-eight-year-old favored a relaxed, start-up look: khakis, button-downs, and Converse All-Stars.
Schrum felt immediate strain, however. He hadn't told anyone, but he was dealing with intense pain in his hands and joints, a condition that later would be diagnosed as degenerative arthritis. Soon Schrum couldn't bend two fingers on his left hand, making lab work difficult. He joined a drug trial, but the medicine proved useless. Schrum tried corticosteroid injections and anti-inflammatory drugs, but his left hand ached, restricting his experiments.
"It just wasn't useful," Schrum says, referring to his tender hand.*
He persisted, nonetheless. Each day in the fall of 2010, Schrum walked through double air-locked doors into a sterile "clean room" before entering a basement laboratory, in the bowels of an office in Cambridge's Kendall Square neighborhood, where he worked deep into the night. Schrum searched for potential modifications of mRNA nucleosides, hoping they might enable the molecule to produce proteins. Like all such rooms, there were no windows, so Schrum had to check a clock to know if it was day or night. A colleague came to visit once in a while, but most of the time, Schrum was alone.
Some of Moderna's founders doubted Schrum could find success and they worried if their venture was doomed from the start. An established MIT scientist turned down a job with the start-up to join pharmaceutical giant Novartis, dubious of Moderna's approach. Colleagues wondered if mRNA could produce proteins, at least on a consistent basis.
As Schrum began testing the modifications in January 2011, he made an unexpected discovery. Karikó and Weissman saw that by turned one of the building blocks for mRNA, a ribonucleoside called uridine, into a slightly different form called pseudouridine, the cell's immune system ignored the mRNA and the molecule avoided an immune response. After a series of experiments in the basement lab, Schrum discovered that a variant of pseudouridine called N1- methyl-pseudouridine did an even better job reducing the cell's innate immune response. Schrum's nucleoside switch enabled even higher protein production than Karikó and Weissman had generated, and Schrum's mRNAs lasted longer than either unmodified molecules or the modified mRNA the Penn academics had used, startling the young researcher. Working alone in a dreary basement and through intense pain, he had actually improved on the Penn professors' work.
Years later, Karikó and Weissman who would win acclaim. In September 2021, the scientists were awarded the Lasker-DeBakey Clinical Medical Research Award. Some predict they eventually will win a Nobel prize. But it would be Schrum's innovation that would form the backbone of both Moderna and Pfizer-BioNTech's Covid-19 vaccine, not the chemical modifications that Karikó and Weissman developed. For Schrum, necessity had truly been the mother of invention.
The Scientist Who Solved Delivery: Kerry Benenato
For several years, Moderna would make slow progress developing drugs to treat various diseases. Eventually, the company decided that mRNA was likely better suited for vaccines. By 2017, Moderna and the National Institutes of Health were discussing working together to develop mRNA–based vaccines, a partnership that buoyed Moderna's executives. There remained a huge obstacle in Moderna's way, however. It was up to Kerry Benenato to find a solution.
Benenato received an early hint of the hurdle in front of her three years earlier, when the organic chemist was first hired. When a colleague gave her a company tour, she was introduced to Moderna's chief scientific officer, Joseph Bolen, who seemed unusually excited to meet her.
"Oh, great!" Bolen said with a smile. "She's the one who's gonna solve delivery."
Bolen gave a hearty laugh and walked away, but Benenato detected seriousness in his quip.
Solve delivery?
It was a lot to expect from a 37-year-old scientist already dealing with insecurities and self-doubt. Benenato was an accomplished researcher who most recently had worked at AstraZeneca after completing post-doctoral studies at Harvard University. Despite her impressive credentials, Benenato battled a lack of confidence that sometimes got in her way. Performance reviews from past employers had been positive, but they usually produced similar critiques: Be more vocal. Do a better job advocating for your ideas. Give us more, Kerry.
Benenato was petite and soft-spoken. She sometimes stuttered or relied on "ums" and "ahs" when she became nervous, especially in front of groups, part of why she sometimes didn't feel comfortable speaking up.
"I'm an introvert," she says. "Self-confidence is something that's always been an issue."
To Benenato, Moderna's vaccine approach seemed promising—the team was packaging mRNAs in microscopic fatty-acid compounds called lipid nanoparticles, or LNPs, that protected the molecules on their way into cells. Moderna's shots should have been producing ample and long-lasting proteins. But the company's scientists were alarmed—they were injecting shots deep into the muscle of mice, but their immune systems were mounting spirited responses to the foreign components of the LNPs, which had been developed by a Canadian company.
This toxicity was a huge issue: A vaccine or drug that caused sharp pain and awful fevers wasn't going to prove very popular. The Moderna team was in a bind: Its mRNA had to be wrapped in the fatty nanoparticles to have a chance at producing plentiful proteins, but the body wasn't tolerating the microscopic encasements, especially upon repeated dosing.
The company's scientists had done everything they could to try to make the molecule's swathing material disappear soon after entering the cells, in order to avoid the unfortunate side effects, such as chills and headaches, but they weren't making headway. Frustration mounted. Somehow, the researchers had to find a way to get the encasements—made of little balls of fat, cholesterol, and other substances—to deliver their payload mRNA and then quickly vanish, like a parent dropping a teenager off at a party, to avoid setting off the immune system in unpleasant ways, even as the RNA and the proteins the molecule created stuck around.
Benenato wasn't entirely shocked by the challenges Moderna was facing. One of the reasons she had joined the upstart company was to help develop its delivery technology. She just didn't realize how pressing the issue was, or how stymied the researchers had become. Benenato also didn't know that Moderna board members were among those most discouraged by the delivery issue. In meetings, some of them pointed out that pharmaceutical giants like Roche Holding and Novartis had worked on similar issues and hadn't managed to develop lipid nanoparticles that were both effective and well tolerated by the body. Why would Moderna have any more luck?
Stephen Hoge insisted the company could yet find a solution.
"There's no way the only innovations in LNP are going to come from some academics and a small Canadian company," insisted Hoge, who had convinced the executives that hiring Benenato might help deliver an answer.
Benenato realized that while Moderna might have been a hot Boston-area start- up, it wasn't set up to do the chemistry necessary to solve their LNP problem. Much of its equipment was old or secondhand, and it was the kind used to tinker with mRNAs, not lipids.
"It was scary," she says.
When Benenato saw the company had a nuclear magnetic resonance spectrometer, which allows chemists to see the molecular structure of material, she let out a sigh of relief. Then Benenato inspected the machine and realized it was a jalopy. The hulking, aging instrument had been decommissioned and left behind by a previous tenant, too old and banged up to bring with them.
Benenato began experimenting with different chemical changes for Moderna's LNPs, but without a working spectrometer she and her colleagues had to have samples ready by noon each day, so they could be picked up by an outside company that would perform the necessary analysis. After a few weeks, her superiors received an enormous bill for the outsourced work and decided to pay to get the old spectrometer running again.
After months of futility, Benenato became impatient. An overachiever who could be hard on herself, she was eager to impress her new bosses. Benenato felt pressure outside the office, as well. She was married with a preschool-age daughter and an eighteen-month-old son. In her last job, Benenato's commute had been a twenty-minute trip to Astra-Zeneca's office in Waltham, outside Boston; now she was traveling an hour to Moderna's Cambridge offices. She became anxious—how was she going to devote the long hours she realized were necessary to solve their LNP quandary while providing her children proper care? Joining Moderna was beginning to feel like a possible mistake.
She turned to her husband and father for help. They reminded her of the hard work she had devoted to establishing her career and said it would be a shame if she couldn't take on the new challenge. Benenato's husband said he was happy to stay home with the kids, alleviating some of her concerns.
Back in the office, she got to work. She wanted to make lipids that were easier for the body to chop into smaller pieces, so they could be eliminated by the body's enzymes. Until then, Moderna, like most others, relied on all kinds of complicated chemicals to hold its LNP packaging together. They weren't natural, though, so the body was having a hard time breaking them down, causing the toxicity.
Benenato began experimenting with simpler chemicals. She inserted "ester bonds"—compounds referred to in chemical circles as "handles" because the body easily grabs them and breaks them apart. Ester bonds had two things going for them: They were strong enough to help ensure the LNP remained stable, acting much like a drop of oil in water, but they also gave the body's enzymes something to target and break down as soon as the LNP entered the cell, a way to quickly rid the body of the potentially toxic LNP components. Benenato thought the inclusion of these chemicals might speed the elimination of the LNP delivery material.
This idea, Benenato realized, was nothing more than traditional, medicinal chemistry. Most people didn't use ester bonds because they were pretty unsophisticated. But, hey, the tricky stuff wasn't working, so Benenato thought she'd see if the simple stuff worked.
Benenato also wanted to try to replace a group of unnatural chemicals in the LNP that was contributing to the spirited and unwelcome response from the immune system. Benenato set out to build a new and improved chemical combination. She began with ethanolamine, a colorless, natural chemical, an obvious start for any chemist hoping to build a more complex chemical combination. No one relied on ethanolamine on its own.
Benenato was curious, though. What would happen if she used just these two simple modifications to the LNP: ethanolamine with the ester bonds? Right away, Benenato noticed her new, super-simple compound helped mRNA create some protein in animals. It wasn't much, but it was a surprising and positive sign. Benenato spent over a year refining her solution, testing more than one hundred variations, all using ethanolamine and ester bonds, showing improvements with each new version of LNP. After finishing her 102nd version of the lipid molecule, which she named SM102, Benenato was confident enough in her work to show it to Hoge and others.
They immediately got excited. The team kept tweaking the composition of the lipid encasement. In 2017, they wrapped it around mRNA molecules and injected the new combination in mice and then monkeys. They saw plentiful, potent proteins were being produced and the lipids were quickly being eliminated, just as Benenato and her colleagues had hoped. Moderna had its special sauce.
That year, Benenato was asked to deliver a presentation to Stephane Bancel, Moderna's chief executive, Afeyan, and Moderna's executive committee to explain why it made sense to use the new, simpler LNP formulation for all its mRNA vaccines. She still needed approval from the executives to make the change. Ahead of the meeting, she was apprehensive, as some of her earlier anxieties returned. But an unusual calm came over her as she began speaking to the group. Benenato explained how experimenting with basic, overlooked chemicals had led to her discovery.
She said she had merely stumbled onto the company's solution, though her bosses understood the efforts that had been necessary for the breakthrough. The board complimented her work and agreed with the idea of switching to the new LNP. Benenato beamed with pride.
"As a scientist, serendipity has been my best friend," she told the executives.
Over the next few years, Benenato and her colleagues would improve on their methods and develop even more tolerable and potent LNP encasement for mRNA molecules. Their work enabled Moderna to include higher doses of vaccine in its shots. In early 2020, Moderna developed Covid-19 shots that included 100 micrograms of vaccine, compared with 30 micrograms in the Pfizer-BioNTech vaccine. That difference appears to help the Moderna vaccine generate higher titers and provide more protection.
"You set out in a career in drug discovery to want to make a difference," Benenato says. "Seeing it come to reality has been surreal and emotional."
Editor's Note: This essay is excerpted from A SHOT TO SAVE THE WORLD: The Inside Story of the Life-or-Death Race for a COVID-19 Vaccine by Gregory Zuckerman, now on sale from Portfolio/Penguin.
*Jason Schrum's arthritis is now in complete remission, thanks to Humira (adalimumab), a TNF-alpha blocker.
Can tech help prevent the insect apocalypse?
Declining numbers of insects, coupled with climate change, can have devastating effects for people in more ways than one. But clever use of technologies like AI could keep them buzzing.
This article originally appeared in One Health/One Planet, a single-issue magazine that explores how climate change and other environmental shifts are making us more vulnerable to infectious diseases by land and by sea - and how scientists are working on solutions.
On a warm summer day, forests, meadows, and riverbanks should be abuzz with insects—from butterflies to beetles and bees. But bugs aren’t as abundant as they used to be, and that’s not a plus for people and the planet, scientists say. The declining numbers of insects, coupled with climate change, can have devastating effects for people in more ways than one. “Insects have been around for a very long time and can live well without humans, but humans cannot live without insects and the many services they provide to us,” says Philipp Lehmann, a researcher in the Department of Zoology at Stockholm University in Sweden. Their decline is not just bad, Lehmann adds. “It’s devastating news for humans.
”Insects and other invertebrates are the most diverse organisms on the planet. They fill most niches in terrestrial and aquatic environments and drive ecosystem functions. Many insects are also economically vital because they pollinate crops that humans depend on for food, including cereals, vegetables, fruits, and nuts. A paper published in PNAS notes that insects alone are worth more than $70 billion a year to the U.S. economy. In places where pollinators like honeybees are in decline, farmers now buy them from rearing facilities at steep prices rather than relying on “Mother Nature.”
And because many insects serve as food for other species—bats, birds and freshwater fish—they’re an integral part of the ecosystem’s food chain. “If you like to eat good food, you should thank an insect,” says Scott Hoffman Black, an ecologist and executive director of the Xerces Society for Invertebrate Conservation in Portland, Oregon. “And if you like birds in your trees and fish in your streams, you should be concerned with insect conservation.”
Deforestation, urbanization, and agricultural spread have eaten away at large swaths of insect habitat. The increasingly poorly controlled use of insecticides, which harms unintended species, and the proliferation of invasive insect species that disrupt native ecosystems compound the problem.
“There is not a single reason why insects are in decline,” says Jessica L. Ware, associate curator in the Division of Invertebrate Zoology at the American Museum of Natural History in New York, and president of the Entomological Society of America. “There are over one million described insect species, occupying different niches and responding to environmental stressors in different ways.”
Jessica Ware, an entomologist at the American Museum of Natural History, is using DNA methods to monitor insects.
Credit:D.Finnin/AMNH
In addition to habitat loss fueling the decline in insect populations, the other “major drivers” Ware identified are invasive species, climate change, pollution, and fluctuating levels of nitrogen, which play a major role in the lifecycle of plants, some of which serve as insect habitants and others as their food. “The causes of world insect population declines are, unfortunately, very easy to link to human activities,” Lehmann says.
Climate change will undoubtedly make the problem worse. “As temperatures start to rise, it can essentially make it too hot for some insects to survive,” says Emily McDermott, an assistant professor in the Department of Entomology and Plant Pathology at the University of Arkansas. “Conversely in other areas, it could potentially also allow other insects to expand their ranges.”
Without Pollinators Humans Will Starve
We may not think much of our planet’s getting warmer by only one degree Celsius, but it can spell catastrophe for many insects, plants, and animals, because it’s often accompanied by less rainfall. “Changes in precipitation patterns will have cascading consequences across the tree of life,” says David Wagner, a professor of ecology and evolutionary biology at the University of Connecticut. Insects, in particular, are “very vulnerable” because “they’re small and susceptible to drying.”
For instance, droughts have put the monarch butterfly at risk of being unable to find nectar to “recharge its engine” as it migrates from Canada and New England to Mexico for winter, where it enters a hibernation state until it journeys back in the spring. “The monarch is an iconic and a much-loved insect,” whose migration “is imperiled by climate change,” Wagner says.
Warming and drying trends in the Western United States are perhaps having an even more severe impact on insects than in the eastern region. As a result, “we are seeing fewer individual butterflies per year,” says Matt Forister, a professor of insect ecology at the University of Nevada, Reno.
There are hundreds of butterfly species in the United States and thousands in the world. They are pollinators and can serve as good indicators of other species’ health. “Although butterflies are only one group among many important pollinators, in general we assume that what’s bad for butterflies is probably bad for other insects,” says Forister, whose research focuses on butterflies. Climate change and habitat destruction are wreaking havoc on butterflies as well as plants, leading to a further indirect effect on caterpillars and butterflies.
Different insect species have different levels of sensitivity to environmental changes. For example, one-half of the bumblebee species in the United States are showing declines, whereas the other half are not, says Christina Grozinger, a professor of entomology at the Pennsylvania State University. Some species of bumble bees are even increasing in their range, seemingly resilient to environmental changes. But other pollinators are dwindling to the point that farmers have to buy from the rearing facilities, which is the case for the California almond industry. “This is a massive cost to the farmer, which could be provided for free, in case the local habitats supported these pollinators,” Lehmann says.
For bees and other insects, climate change can harm the plants they depend on for survival or have a negative impact on the insects directly. Overly rainy and hot conditions may limit flowering in plants or reduce the ability of a pollinator to forage and feed, which then decreases their reproductive success, resulting in dwindling populations, Grozinger explains.
“Nutritional deprivation can also make pollinators more sensitive to viruses and parasites and therefore cause disease spread,” she says. “There are many ways that climate change can reduce our pollinator populations and make it more difficult to grow the many fruit, vegetable and nut crops that depend on pollinators.”
Disease-Causing Insects Can Bring More Outbreaks
While some much-needed insects are declining, certain disease-causing species may be spreading and proliferating, which is another reason for human concern. Many mosquito types spread malaria, Zika virus, West Nile virus, and a brain infection called equine encephalitis, along with other diseases as well as heartworms in dogs, says Michael Sabourin, president of the Vermont Entomological Society. An animal health specialist for the state, Sabourin conducts vector surveys that identify ticks and mosquitoes.
Scientists refer to disease-carrying insects as vector species and, while there’s a limited number of them, many of these infections can be deadly. Fleas were a well-known vector for the bubonic plague, while kissing bugs are a vector for Chagas disease, a potentially life-threatening parasitic illness in humans, dogs, and other mammals, Sabourin says.
As the planet heats up, some of the creepy crawlers are able to survive milder winters or move up north. Warmer temperatures and a shorter snow season have spawned an increasing abundance of ticks in Maine, including the blacklegged tick (Ixodes scapularis), known to transmit Lyme disease, says Sean Birkel, an assistant professor in the Climate Change Institute and Cooperative Extension at the University of Maine.
Coupled with more frequent and heavier precipitation, rising temperatures bring a longer warm season that can also lead to a longer period of mosquito activity. “While other factors may be at play, climate change affects important underlying conditions that can, in turn, facilitate the spread of vector-borne disease,” Birkel says.
For example, if mosquitoes are finding fewer of their preferred food sources, they may bite humans more. Both male and female mosquitoes feed on sugar as part of their normal behavior, but if they aren’t eating their fill, they may become more bloodthirsty. One recent paper found that sugar-deprived Anopheles gambiae females go for larger blood meals to stay in good health and lay eggs. “More blood meals equals more chances to pick up and transmit a pathogen,” McDermott says, He adds that climate change could reduce the number of available plants to feed on. And while most mosquitoes are “generalist sugar-feeders” meaning that they will likely find alternatives, losing their favorite plants can make them hungrier for blood.
Similar to the effect of losing plants, mosquitoes may get turned onto people if they lose their favorite animal species. For example, some studies found that Culex pipiens mosquitoes that transmit the West Nile virus feed primarily on birds in summer. But that changes in the fall, at least in some places. Because there are fewer birds around, C. pipiens switch to mammals, including humans. And if some disease-carrying insect species proliferate or increase their ranges, that increases chances for human infection, says McDermott. “A larger concern is that climate change could increase vector population sizes, making it more likely that people or animals would be bitten by an infected insect.”
Science Can Help Bring Back the Buzz
To help friendly insects thrive and keep the foes in check, scientists need better ways of trapping, counting, and monitoring insects. It’s not an easy job, but artificial intelligence and molecular methods can help. Ware’s lab uses various environmental DNA methods to monitor freshwater habitats. Molecular technologies hold much promise. The so-called DNA barcodes, in which species are identified using a short string of their genes, can now be used to identify birds, bees, moths and other creatures, and should be used on a larger scale, says Wagner, the University of Connecticut professor. “One day, something akin to Star Trek’s tricorder will soon be on sale down at the local science store.”
Scientists are also deploying artificial intelligence, or AI, to identify insects in agricultural systems and north latitudes where there are fewer bugs, Wagner says. For instance, some automated traps already use the wingbeat frequencies of mosquitoes to distinguish the harmless ones from the disease-carriers. But new technology and software are needed to further expand detection based on vision, sound, and odors.
“Because of their ubiquity, enormity of numbers, and seemingly boundless diversity, we desperately need to develop molecular and AI technologies that will allow us to automate sampling and identification,” says Wagner. “That would accelerate our ability to track insect populations, alert us to the presence of new disease vectors, exotic pest introductions, and unexpected declines.”
Your surgery could harm yourself and the planet. Here's what some doctors are doing about it.
Certain gases used for anesthesia are 3,000 times more damaging for the climate than CO2. Some anesthesiologists are pointing to other solutions.
This is part 1 of a three part series on a new generation of doctors leading the charge to make the health care industry more sustainable - for the benefit of their patients and the planet. Read part 2 here and part 3 here.
Susanne Koch, an anesthesiologist and neurologist, reached a pivot point when she was up to her neck in water, almost literally. The basement of her house in Berlin had flooded in the summer of 2018, when Berlin was pummeled by unusually strong rains. After she drained the house, “I wanted to dig into facts, to understand how exactly these extreme weather events are related to climate change,” she says.
Studying the scientific literature, she realized how urgent the climate crisis is, but the biggest shock was to learn that her profession contributed substantially to the problem: Inhalation gases used during medical procedures are among the most damaging greenhouse gases. Some inhalation gases are 3,000 times more damaging for the climate than CO2, Koch discovered. “Spending seven hours in the surgery room is the equivalent of driving a car for four days nonstop,” she says. Her job of helping people at Europe’s largest university hospital, the Charité in Berlin, was inadvertently damaging both the people and the planet.
“Nobody had ever even mentioned a word about that during my training,” Koch says.
On the whole, the medical sector is responsible for a disproportionally large percentage of greenhouse gas emissions, with the U.S. as the biggest culprit. According to a key paper published in 2020 in Health Affairs, the health industry “is among the most carbon-intensive service sectors in the industrialized world,” accounting for between 4.4 percent and 4.6 percent of greenhouse gas emissions. “It’s not just anesthesia but health care that has a problem,” says Jodi Sherman, anesthesiology professor and Medical Director of the Program on Healthcare Environmental Sustainability at Yale University as well as co-director of the Lancet Planetary Health Commission on Sustainable Healthcare. In the U.S., health care greenhouse gas emissions make up about 8.5 percent of domestic greenhouse gas emissions. They rose 6 percent from 2010 to 2018, to nearly 1,700 kilograms per person, more than in any other nation.
Of course, patients worry primarily about safety, not sustainability. Yet, Koch emphasizes that “as doctors, we have the responsibility to do no harm, and this includes making sure that we use resources as sustainably as possible.” Studies show that 2018 greenhouse gas and toxic air pollutant emissions resulted in the loss of 388,000 disability-adjusted life years in the U.S. alone. “Disease burden from health care pollution is of the same order of magnitude as deaths from preventable medical errors, and should be taken just as seriously,” Sherman cautions.
When Koch, the anesthesiologist, started discussing sustainable options with colleagues, the topic was immediately met with plenty of interest. Her experience is consistent with the latest representative poll of the nonprofit Foundation Health in Germany. Nine out of ten doctors were interested in urgently finding sustainable solutions for medical services but lacked knowhow and resources. For teaching purposes, Sherman and her team have developed the Yale Gassing Greener app that allows anesthesiologists to compare how much pollution they can avoid through choosing different anesthesia methods. Sherman also published professional guidelines intended to help her colleagues better understand how various methods affect carbon emissions.
Significant traces of inhalation gases have been found in Antarctica and the Himalayas, far from the vast majority of surgery rooms.
A solution espoused by both Sherman and Koch is comparatively simple: They stopped using desflurane, which is by far the most damaging of all inhalation gases to the climate. Its greenhouse effect is 2,590 times stronger than carbon dioxide. The Yale New Haven Hospital already stopped using desflurane in 2013, becoming the first known healthcare organization to eliminate a drug based on environmental grounds. Sherman points out that this resulted in saving more than $1.2 million in costs and 1,600 tons of CO2 equivalents, about the same as the exhaust from 360 passenger vehicles per year.
At the Charité, Koch claims that switching to other anesthesiology choices, such as propofol, has eliminated 90 percent of the climate gas emissions in the anesthesiology department since 2016. Young anesthesiologists are still taught to use desflurane as the standard because desflurane is absorbed less into the patients’ bodies, and they wake up faster. However, Koch who has worked as an anesthesiologist since 2006, says that with a little bit of experience, you can learn when to stop giving the propofol so it's timed just as well with a person’s wake-up process. In addition, “patients are less likely to feel nauseous after being given propofol,” Koch says. Intravenous drugs might require more skill, she adds, "but there is nothing unique to the drug desflurane that cannot be accomplished with other medications.”
Desflurane isn’t the only gas to be concerned about. Nitrous oxide is the second most damaging because it’s extremely long-lived in the environment, and it depletes the ozone layer. Climate-conscious anesthesiologists are phasing out this gas, too, or have implemented measures to decrease leaks.
Internationally, 192 governments agreed in the Kyoto protocol of 2005 to reduce halogenated hydrocarbons – resulting from inhalation gases, including desflurane and nitrous oxide – because of their immense climate-warming potential, and in 2016, they pledged to eliminate them by 2035. However, the use of inhalation anesthetics continues to increase worldwide, not least because more people access healthcare in developing countries, and because people in industrialized countries live longer and therefore need more surgeries. Significant traces of inhalation gases have been found in Antarctica and the Himalayas, far from the vast majority of surgery rooms.
Certain companies are now pushing new technology to capture inhalation gases before they are released into the atmosphere, but both Sherman and Koch believe marketing claims of 99 percent efficiency amount to greenwashing. After investigating the technology first-hand and visiting the company that is producing such filters in Germany, Koch concluded that such technology only reduces emissions by 25 percent. And Sherman believes such initiatives are akin to the fallacy of recycling plastic. In addition to questioning their efficiency, Sherman fears such technology “gives the illusion there is a magical solution that means I don’t need to change my behavior, reduce my waste and choose less harmful options.”
Financial interests are at play, too. “Desflurane is the most expensive inhalation gas, and some think, the most expensive must be the best,” Koch says. Both Koch and Sherman lament that efforts to increase sustainability in the medical sector are entirely voluntary in their countries and led by a few dedicated individual professionals while industry-wide standards and transparency are needed, a notion expressed in the American Hospital Association’s Sustainability Roadmap.
Susanne Koch, an anesthesiologist in Berlin, wants her colleagues to stop using a gas called desflurane, which is by far the most damaging of all inhalation gases to the climate.
Adobe Stock
Other countries have done more. The European Union recommends reducing inhalation gases and even contemplated a ban of desflurane, except in medical emergencies. In 2008, the National Health Service (NHS) created a Sustainable Development Unit, which measures CO2 emissions in the U.K. health sector. NHS is the first national health service that pledged to reach net zero carbon by 2040. The carbon footprint of the NHS fell by 26 percent from 1990 to 2019, mostly due to reduced use of certain inhalers and the switch to renewable energy for heat and power. “The evidence that the climate emergency is a health emergency is overwhelming,” said Nick Watts, the NHS Chief Sustainability Officer, in a press release, “with health professionals already needing to manage its symptoms.”
Sherman is a leading voice in demanding action in the U.S. To her, comprehensive solutions start with the mandatory, transparent measurement of emissions in the health sector to tackle the biggest sources of pollution. While the Biden administration highlighted its efforts to reduce these kinds of emissions during the United Nations Climate Conference (COP27) in November 2022 and U.S. delegates announced that more than 100 health care organizations signed the voluntary Health Sector Climate Pledge, with the aim to reduce emissions by 50 percent in the next eight years, Sherman is convinced that voluntary pledges are not enough. “Voluntary measures are insufficient,” she testified in congress. “The vast majority of U.S. health care organizations remain uncommitted to timely action. Those that are committed lack policies and knowledge to support necessary changes; even worse, existing policies drive inappropriate consumption of resources and pollution.”
Both Sherman and Koch look at the larger picture. “Health care organizations have an obligation to their communities to protect public health,” Sherman says. “We must lead by example. That includes setting ambitious, science-based carbon reduction targets to achieve net zero emissions before 2050. We must quantify current emissions and their sources, particularly throughout the health care supply chains.”