This Special Music Helped Preemie Babies’ Brains Develop
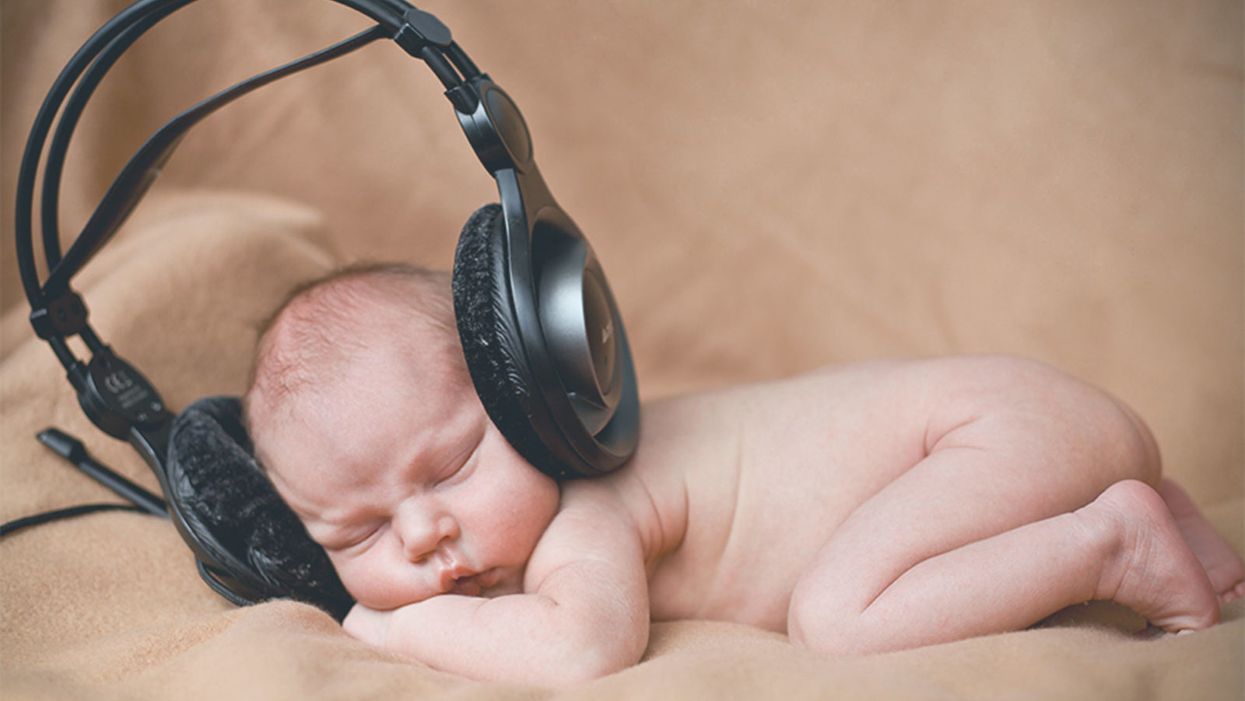
Listening to music helped preterm babies' brains develop, according to the results of a new Swiss study.
Move over, Baby Einstein: New research from Switzerland shows that listening to soothing music in the first weeks of life helps encourage brain development in preterm babies.
For the study, the scientists recruited a harpist and a new-age musician to compose three pieces of music.
The Lowdown
Children who are born prematurely, between 24 and 32 weeks of pregnancy, are far more likely to survive today than they used to be—but because their brains are less developed at birth, they're still at high risk for learning difficulties and emotional disorders later in life.
Researchers in Geneva thought that the unfamiliar and stressful noises in neonatal intensive care units might be partially responsible. After all, a hospital ward filled with alarms, other infants crying, and adults bustling in and out is far more disruptive than the quiet in-utero environment the babies are used to. They decided to test whether listening to pleasant music could have a positive, counterbalancing effect on the babies' brain development.
Led by Dr. Petra Hüppi at the University of Geneva, the scientists recruited Swiss harpist and new-age musician Andreas Vollenweider (who has collaborated with the likes of Carly Simon, Bryan Adams, and Bobby McFerrin). Vollenweider developed three pieces of music specifically for the NICU babies, which were played for them five times per week. Each track was used for specific purposes: To help the baby wake up; to stimulate a baby who was already awake; and to help the baby fall back asleep.
When they reached an age equivalent to a full-term baby, the infants underwent an MRI. The researchers focused on connections within the salience network, which determines how relevant information is, and then processes and acts on it—crucial components of healthy social behavior and emotional regulation. The neural networks of preemies who had listened to Vollenweider's pieces were stronger than preterm babies who had not received the intervention, and were instead much more similar to full-term babies.
Next Up
The first infants in the study are now 6 years old—the age when cognitive problems usually become diagnosable. Researchers plan to follow up with more cognitive and socio-emotional assessments, to determine whether the effects of the music intervention have lasted.
The first infants in the study are now 6 years old—the age when cognitive problems usually become diagnosable.
The scientists note in their paper that, while they saw strong results in the babies' primary auditory cortex and thalamus connections—suggesting that they had developed an ability to recognize and respond to familiar music—there was less reaction in the regions responsible for socioemotional processing. They hypothesize that more time spent listening to music during a NICU stay could improve those connections as well; but another study would be needed to know for sure.
Open Questions
Because this initial study had a fairly small sample size (only 20 preterm infants underwent the musical intervention, with another 19 studied as a control group), and they all listened to the same music for the same amount of time, it's still undetermined whether variations in the type and frequency of music would make a difference. Are Vollenweider's harps, bells, and punji the runaway favorite, or would other styles of music help, too? (Would "Baby Shark" help … or hurt?) There's also a chance that other types of repetitive sounds, like parents speaking or singing to their children, might have similar effects.
But the biggest question is still the one that the scientists plan to tackle next: Whether the intervention lasts as the children grow up. If it does, that's great news for any family with a preemie — and for the baby-sized headphone industry.
This “Absolutely Tireless” Researcher Made an Important Breakthrough for Cancer Patients
Theo Roth.
After months of looking at dead cells under a microscope, Theo Roth finally glimpsed what he had been hoping to see—flickers of green. His method was working.
"If we can go into the cell and add in new code and instructions, now we can give it whatever new functions we want."
When Roth joined the laboratory of Alex Marson at the University of California, San Francisco in June 2016, he set to work trying to figure out a new way to engineer human T cells, a type of white blood cell that's an important part of the immune system. If he succeeded, the resulting approach could make it easier and faster for scientists to develop and test cell and gene therapies, new treatments that involve genetically reprogramming the body's own cells.
For decades, researchers have been using engineered viruses to bestow human cells with new genetic characteristics. These so-called viral vectors "infect" human cells, transferring whatever new genetic material scientists put into them. The idea is that this new DNA could give T cells a boost to better fight diseases like cancer and HIV.
Several successful clinical trials have used virally-modified human T cells, and in fact, the U.S. Food and Drug Administration last year approved two such groundbreaking cancer gene therapies, Kymriah and Yescarta. But the process of genetically manipulating cells with viruses is expensive and time-consuming. In addition, viruses tend to randomly insert DNA with little predictability.
"What Theo wanted to do was to paste in big sequences of DNA at a targeted site without viruses," says Marson, an associate professor of microbiology and immunology. "That would have the benefit of being able to rewrite a specific site in the genome and do it flexibly and quickly without having to make a new virus for every site you want to manipulate."
Scientists have for a while been interested in non-viral engineering methods, but T cells are fragile and notoriously difficult to work with.
Previously, Marson's lab had collaborated with CRISPR pioneer Jennifer Doudna and her team at the University of California, Berkeley to use an electrical pulse together with CRISPR components to knock out certain genes. They also found some success with inserting very small pieces of DNA into a targeted site.
But Roth, a 27-year-old graduate student at UCSF pursuing MD and PhD degrees, was determined to figure out how to paste in much bigger sequences of genetic information. Marson says it was an "ambitious" goal. Scientists had tried before, but found that stuffing large chunks of DNA into T cells would quickly kill them.
"If we can go into the cell and add in new code and instructions, now we can give it whatever new functions we want," Roth says. "If you can add in new DNA sequences at the site that you want, then you have a much greater capacity to generate a cell that's going to be therapeutic or curative for a disease."
"He has already made his mark on the field."
So Roth began experimenting with hundreds of different variables a week, trying to find the right conditions to allow him to engineer T cells without the need for viruses. To know if the technique was working, Roth and his colleagues used a green fluorescent protein that would be expressed in cells that had successfully been modified.
"We went from having a lot of dead cells that didn't have any green to having maybe 1 percent of them being green," Roth says. "At that stage we got really excited."
After nearly a year of testing, he and collaborators found a combination of T cell ratios and DNA quantity mixed with CRISPR and zaps of electricity that seemed to work. These electrical pulses, called electroporation, deliver a jolt to cells that makes their membranes temporarily more permeable, allowing the CRISPR system to slip through. Once inside cells, CRISPR seeks out a specific place in the genome and makes a programmed, precise edit.
Roth and his colleagues used the approach to repair a genetic defect in T cells taken from children with a rare autoimmune disease and also to supercharge T cells so that they'd seek out and selectively kill human cancer cells while leaving healthy cells intact. In mice transplanted with human melanoma tissue, the edited T cells went to straight to the cancerous cells and attacked them. The findings were published in Nature in July.
Marson and Roth think even a relatively small number of modified T cells could be effective at treating some cancers, infections, and autoimmune diseases.
Roth is now working with the Parker Institute for Cancer Immunotherapy in San Francisco to engineer cells to treat a variety of cancers and hopefully commercialize his technique. Fred Ramsdell, vice president at the Parker Institute, says he's impressed by Roth's work. "He has already made his mark on the field."
Right now, there's a huge manufacturing backlog for viruses. If researchers want to start a clinical trial to test a new gene or cell therapy, they often have to wait a year to get the viruses they need.
"I think the biggest immediate impact is that it will lower the cost of a starting an early phase clinical trial."
Ramsdell says what Roth's findings allow researchers to do is engineer T cells quickly and more efficiently, cutting the time it takes to make them from several months to just a few weeks. That will allow researchers to develop and test several potential therapies in the lab at once.
"I think the biggest immediate impact is that it will lower the cost of a starting an early phase clinical trial," Roth says.
This isn't the first time Roth's work has been in the spotlight. As an undergraduate at Stanford University, he made significant contributions to traumatic brain injury research by developing a mouse model for observing the brain's cellular response to a concussion. He started the research, which was also published in Nature, the summer before entering college while he was an intern in Dorian McGavern's lab at the National Institutes of Health.
When Roth entered UCSF as a graduate student, his scientific interests shifted.
"It's definitely a big leap" from concussion research, says McGavern, who still keeps in touch with Roth. But he says he's not surprised about Roth's path. "He's absolutely tireless when it comes to the pursuit of science."
Roth says he's optimistic about the potential for gene and cell therapies to cure patients. "I want to try to figure out what one of the next therapies we should put into patients should be."
A patient with below-knee AMI amputation walks up the stairs.
"Here's a question for you," I say to our dinner guests, dodging a knowing glance from my wife. "Imagine a future in which you could surgically replace your legs with robotic substitutes that had all the functionality and sensation of their biological counterparts. Let's say these new legs would allow you to run all day at 20 miles per hour without getting tired. Would you have the surgery?"
Why are we so married to the arbitrary distinction between rehabilitating and augmenting?
Like most people I pose this question to, our guests respond with some variation on the theme of "no way"; the idea of undergoing a surgical procedure with the sole purpose of augmenting performance beyond traditional human limits borders on the unthinkable.
"Would your answer change if you had arthritis in your knees?" This is where things get interesting. People think differently about intervention when injury or illness is involved. The idea of a major surgery becomes more tractable to us in the setting of rehabilitation.
Consider the simplistic example of human walking speed. The average human walks at a baseline three miles per hour. If someone is only able to walk at one mile per hour, we do everything we can to increase their walking ability. However, to take a person who is already able to walk at three miles per hour and surgically alter their body so that they can walk twice as fast seems, to us, unreasonable.
What fascinates me about this is that the three-mile-per-hour baseline is set by arbitrary limitations of the healthy human body. If we ignore this reference point altogether, and consider that each case simply offers an improvement in walking ability, the line between augmentation and rehabilitation all but disappears. Why, then, are we so married to this arbitrary distinction between rehabilitating and augmenting? What makes us hold so tightly to baseline human function?
Where We Stand Now
As the functionality of advanced prosthetic devices continues to increase at an astounding rate, questions like these are becoming more relevant. Experimental prostheses, intended for the rehabilitation of people with amputation, are now able to replicate the motions of biological limbs with high fidelity. Neural interfacing technologies enable a person with amputation to control these devices with their brain and nervous system. Before long, synthetic body parts will outperform biological ones.
Our approach allows people to not only control a prosthesis with their brain, but also to feel its movements as if it were their own limb.
Against this backdrop, my colleagues and I developed a methodology to improve the connection between the biological body and a synthetic limb. Our approach, known as the agonist-antagonist myoneural interface ("AMI" for short), enables us to reflect joint movement sensations from a prosthetic limb onto the human nervous system. In other words, the AMI allows people to not only control a prosthesis with their brain, but also to feel its movements as if it were their own limb. The AMI involves a reimagining of the amputation surgery, so that the resultant residual limb is better suited to interact with a neurally-controlled prosthesis. In addition to increasing functionality, the AMI was designed with the primary goal of enabling adoption of a prosthetic limb as part of a patient's physical identity (known as "embodiment").
Early results have been remarkable. Patients with below-knee AMI amputation are better able to control an experimental prosthetic leg, compared to people who had their legs amputated in the traditional way. In addition, the AMI patients show increased evidence of embodiment. They identify with the device, and describe feeling as though it is part of them, part of self.
Where We're Going
True embodiment of robotic devices has the potential to fundamentally alter humankind's relationship with the built world. Throughout history, humans have excelled as tool builders. We innovate in ways that allow us to design and augment the world around us. However, tools for augmentation are typically external to our body identity; there is a clean line drawn between smart phone and self. As we advance our ability to integrate synthetic systems with physical identity, humanity will have the capacity to sculpt that very identity, rather than just the world in which it exists.
For this potential to be realized, we will need to let go of our reservations about surgery for augmentation. In reality, this shift has already begun. Consider the approximately 17.5 million surgical and minimally invasive cosmetic procedures performed in the United States in 2017 alone. Many of these represent patients with no demonstrated medical need, who have opted to undergo a surgical procedure for the sole purpose of synthetically enhancing their body. The ethical basis for such a procedure is built on the individual perception that the benefits of that procedure outweigh its costs.
At present, it seems absurd that amputation would ever reach this point. However, as robotic technology improves and becomes more integrated with self, the balance of cost and benefit will shift, lending a new perspective on what now seems like an unfathomable decision to electively amputate a healthy limb. When this barrier is crossed, we will collide head-on with the question of whether it is acceptable for a person to "upgrade" such an essential part of their body.
At a societal level, the potential benefits of physical augmentation are far-reaching. The world of robotic limb augmentation will be a world of experienced surgeons whose hands are perfectly steady, firefighters whose legs allow them to kick through walls, and athletes who never again have to worry about injury. It will be a world in which a teenage boy and his grandmother embark together on a four-hour sprint through the woods, for the sheer joy of it. It will be a world in which the human experience is fundamentally enriched, because our bodies, which play such a defining role in that experience, are truly malleable.
This is not to say that such societal benefits stand without potential costs. One justifiable concern is the misuse of augmentative technologies. We are all quite familiar with the proverbial supervillain whose nervous system has been fused to that of an all-powerful robot.
The world of robotic limb augmentation will be a world of experienced surgeons whose hands are perfectly steady.
In reality, misuse is likely to be both subtler and more insidious than this. As with all new technology, careful legislation will be necessary to work against those who would hijack physical augmentations for violent or oppressive purposes. It will also be important to ensure broad access to these technologies, to protect against further socioeconomic stratification. This particular issue is helped by the tendency of the cost of a technology to scale inversely with market size. It is my hope that when robotic augmentations are as ubiquitous as cell phones, the technology will serve to equalize, rather than to stratify.
In our future bodies, when we as a society decide that the benefits of augmentation outweigh the costs, it will no longer matter whether the base materials that make us up are biological or synthetic. When our AMI patients are connected to their experimental prosthesis, it is irrelevant to them that the leg is made of metal and carbon fiber; to them, it is simply their leg. After our first patient wore the experimental prosthesis for the first time, he sent me an email that provides a look at the immense possibility the future holds:
What transpired is still slowly sinking in. I keep trying to describe the sensation to people. Then this morning my daughter asked me if I felt like a cyborg. The answer was, "No, I felt like I had a foot."