When Are We Obligated To Edit Wild Creatures?
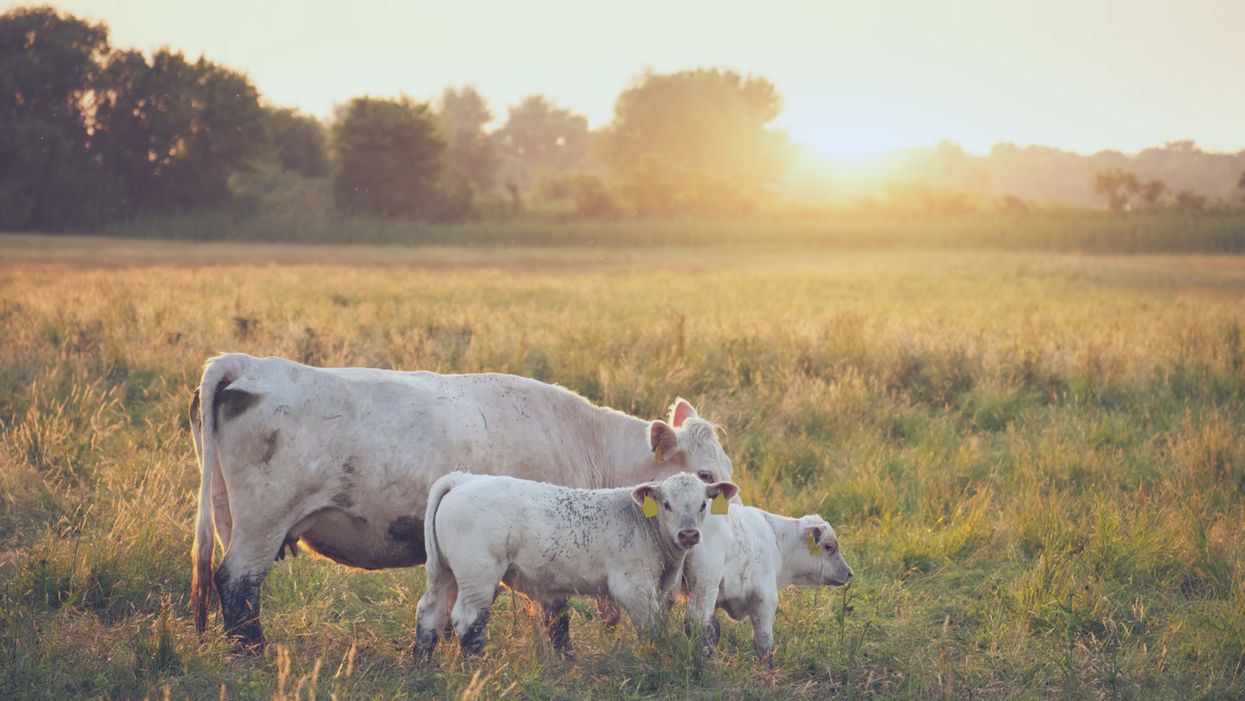
Cows on a pasture, who, among other mammals, could experience immense suffering from the New World screwworm.
Combining CRISPR genome editing with the natural phenomenon of gene drive allows us to rewrite the genomes of wild organisms. The benefits of saving children from malaria by editing mosquitoes are obvious and much discussed, but humans aren't the only creatures who suffer. If we gain the power to intervene in a natural world "red in tooth and claw," yet decline to use it, are we morally responsible for the animal suffering that we could have prevented?
Given the power to alter the workings of the natural world, are we morally obligated to use it?
The scenario that may redefine our relationship with the natural world begins with fine clothing. You're dressed to the nines for a formal event, but you arrived early, and it's such a beautiful day that you decided to take a stroll by the nearby lake. Suddenly, you hear the sound of splashing and screams. A child is drowning! Will you dive in to save them? Or let them die, and preserve your expensive outfit?
The philosopher Peter Singer posited this scenario to show that we are all terrible human beings. Just about everyone would save the child and ruin the outfit... leading Singer to question why so few of us give equivalent amounts of money to save children on the other side of the world. The Against Malaria Foundation averages one life saved for every $7000.
But despite having a local bias, our moral compasses aren't completely broken. You never even considered letting the child drown because the situation wasn't your fault. That's because the cause of the problem simply isn't relevant: as the one who could intervene, the consequences are on your head. We are morally responsible for intervening in situations we did not create.
There is a critical difference between Singer's original scenario and the one above: in his version, it was a muddy pond. Any adult can rescue a child from a muddy pond, but a lake is different; you can only save the child if you know how to swim. We only become morally responsible when we acquire the power to intervene.
Few would disagree with either of these moral statements, but when they are combined with increasingly powerful technologies, the implications are deeply unsettling. Given the power to alter the workings of the natural world, are we morally obligated to use it? Recent developments suggest we had best determine the answer soon because, technologically, we are learning to swim. What choices will we make?
Gene drive is a natural phenomenon that occurs when a genetic element reliably spreads through a population even though it reduces the reproductive fitness of individual organisms. Nature has evolved many different mechanisms that result in gene drive, so many that it's nearly impossible to find an organism that doesn't have at least one driving element somewhere in its genome. More than half of our own DNA comprises the broken remnants of gene drives, plus a few active copies.
Scientists have long dreamed of harnessing gene drive to block mosquito-borne disease, with little success. Then came CRISPR genome editing, which works by cutting target genes and replacing them with a new sequence. What happens if you replace the original sequence with the edited version and an encoded copy of the CRISPR system? Gene drive.
CRISPR is a molecular scalpel that we can use to cut, and therefore replace, just about any DNA sequence in any cell. Encode the instructions for the CRISPR system adjacent to the new sequence, and genome editing will occur in the reproductive cells of subsequent generations of heterozygotes, always converting the original wild-type version to the new edited version. By ensuring that offspring will all be born of one sex, or by arranging for organisms that inherit two copies of the gene drive to be sterile, it's theoretically possible to cause a population crash.
(Credit: Esvelt)
When my colleagues and I first described this technology in 2014, we initially focused on the imperative for early transparency. Gene drive research is more like civic governance than traditional technology development: you can decline a treatment recommended by your doctor, but you can’t opt out when people change the shared environment. Applying the traditional closeted model of science to gene drive actively denies people a voice in decisions intended to affect them - and reforming scientific incentives for gene drive could be the first step to making all of science faster and safer.
But open gene drive research is clearly aligned with virtually all of our values. It's when technology places our deepest moral beliefs in conflict that we struggle, and learn who we truly are.
Two of our strongest moral beliefs include our reverence for the natural world and our abhorrence of suffering. Yet some natural species inherently cause tremendous suffering. Are we morally obligated to alter or even eradicate them?
To anyone who doubts that the natural world can inflict unimaginable suffering, consider the New World screwworm.
Judging by history, the answer depends on who is doing the suffering. We view the eradication of smallpox as one of our greatest triumphs, clearly demonstrating that we value human lives over the existence of disease-causing microorganisms. The same principle holds today for malaria: few would argue against using gene drive to crash populations of malarial mosquitoes to help eradicate the disease. There are more than 3500 species of mosquitoes, only three of which would be affected, and once malaria is gone, the mosquitoes could be allowed to recover. It would be extremely surprising if African nations decided not to eradicate malaria.
The more interesting question concerns our moral obligations to animals in the state of nature.
To anyone who doubts that the natural world can inflict unimaginable suffering, consider the New World screwworm, Cochyliomyia hominivorax. Female screwworm flies lay their eggs in open wounds, generating maggots that devour healthy tissue, gluttonously burrowing into the flesh of their host until they drop, engorged and sated, to metamorphose. Yet before they fall, the maggots in a wound emit a pheromone attracting new females, thereby acting as both conductors and performers in a macabre parade that consumes the host alive. The pain is utterly excruciating, so much so that infested people often require morphine before doctors can even examine the wound. Worst of all, the New World screwworm specializes in devouring complex mammals.
Every second of every day, hundreds of millions of animals suffer the excruciating agony of being eaten alive. It has been so throughout North and South America for millions of years. Until 2001, when humanity eradicated the last screwworm fly north of Panama using the “sterile insect technique�. This was not done to protect wild animals or even people, but for economic reasons: the cost of the program was small relative to the immense damage wrought by the screwworm on North American cattle, sheep, and goats. There were no obvious ecological effects. Despite being almost completely unknown even among animal rights activists, the screwworm elimination campaign may well have been one of the greatest triumphs of animal well-being.
Unfortunately, sterile insect technique isn't powerful enough to eradicate the screwworm from South America, where it is more entrenched and protected by the rougher terrain. But gene drive is.
Contrary to news hype, gene drive alone can't cause extinction, but if combined with conventional measures it might be possible to remove targeted species from the wild. For certain species that cause immense suffering, we may be morally obligated to do just that.
(Credit: Esvelt)
South Americans may well decide to eradicate screwworm for the same economic reasons that it was eradicated from North America: the fly inflicts $4 billion in annual damages on struggling rural communities that can least afford it. It need not go extinct, of course; the existence of the sterile insect facility in Panama proves that we can maintain the screwworm indefinitely in captivity on already dead meat.
Yet if for some reason humanity chooses to leave the screwworm as it is - even for upstanding moral reasons, whatever those may be - the knowledge of our responsibility should haunt us.
Tennyson wrote,
Are God and Nature then at strife,
That Nature lends such evil dreams?
So careful of the type she seems,
So careless of the single life.
Evolution by natural selection cares nothing for the single life, nor suffering, nor euphoria, save for their utility in replication. Theoretically, we do. But how much?
[Editor's Note: This story was originally published in May 2018. We are resurfacing archive hits while our staff is on vacation.]
A movie still from the 1966 film "Fantastic Voyage"
In the 1966 movie "Fantastic Voyage," actress Raquel Welch and her submarine were shrunk to the size of a cell in order to eliminate a blood clot in a scientist's brain. Now, 55 years later, the scenario is becoming closer to reality.
California-based startup Bionaut Labs has developed a nanobot about the size of a grain of rice that's designed to transport medication to the exact location in the body where it's needed. If you think about it, the conventional way to deliver medicine makes little sense: A painkiller affects the entire body instead of just the arm that's hurting, and chemotherapy is flushed through all the veins instead of precisely targeting the tumor.
"Chemotherapy is delivered systemically," Bionaut-founder and CEO Michael Shpigelmacher says. "Often only a small percentage arrives at the location where it is actually needed."
But what if it was possible to send a tiny robot through the body to attack a tumor or deliver a drug at exactly the right location?
Several startups and academic institutes worldwide are working to develop such a solution but Bionaut Labs seems the furthest along in advancing its invention. "You can think of the Bionaut as a tiny screw that moves through the veins as if steered by an invisible screwdriver until it arrives at the tumor," Shpigelmacher explains. Via Zoom, he shares the screen of an X-ray machine in his Culver City lab to demonstrate how the half-transparent, yellowish device winds its way along the spine in the body. The nanobot contains a tiny but powerful magnet. The "invisible screwdriver" is an external magnetic field that rotates that magnet inside the device and gets it to move and change directions.
The current model has a diameter of less than a millimeter. Shpigelmacher's engineers could build the miniature vehicle even smaller but the current size has the advantage of being big enough to see with bare eyes. It can also deliver more medicine than a tinier version. In the Zoom demonstration, the micorobot is injected into the spine, not unlike an epidural, and pulled along the spine through an outside magnet until the Bionaut reaches the brainstem. Depending which organ it needs to reach, it could be inserted elsewhere, for instance through a catheter.
"The hope is that we can develop a vehicle to transport medication deep into the body," says Max Planck scientist Tian Qiu.
Imagine moving a screw through a steak with a magnet — that's essentially how the device works. But of course, the Bionaut is considerably different from an ordinary screw: "At the right location, we give a magnetic signal, and it unloads its medicine package," Shpigelmacher says.
To start, Bionaut Labs wants to use its device to treat Parkinson's disease and brain stem gliomas, a type of cancer that largely affects children and teenagers. About 300 to 400 young people a year are diagnosed with this type of tumor. Radiation and brain surgery risk damaging sensitive brain tissue, and chemotherapy often doesn't work. Most children with these tumors live less than 18 months. A nanobot delivering targeted chemotherapy could be a gamechanger. "These patients really don't have any other hope," Shpigelmacher says.
Of course, the main challenge of the developing such a device is guaranteeing that it's safe. Because tissue is so sensitive, any mistake could risk disastrous results. In recent years, Bionaut has tested its technology in dozens of healthy sheep and pigs with no major adverse effects. Sheep make a good stand-in for humans because their brains and spines are similar to ours.
The Bionaut device is about the size of a grain of rice.
Bionaut Labs
"As the Bionaut moves through brain tissue, it creates a transient track that heals within a few weeks," Shpigelmacher says. The company is hoping to be the first to test a nanobot in humans. In December 2022, it announced that a recent round of funding drew $43.2 million, for a total of 63.2 million, enabling more research and, if all goes smoothly, human clinical trials by early next year.
Once the technique has been perfected, further applications could include addressing other kinds of brain disorders that are considered incurable now, such as Alzheimer's or Huntington's disease. "Microrobots could serve as a bridgehead, opening the gateway to the brain and facilitating precise access of deep brain structure – either to deliver medication, take cell samples or stimulate specific brain regions," Shpigelmacher says.
Robot-assisted hybrid surgery with artificial intelligence is already used in state-of-the-art surgery centers, and many medical experts believe that nanorobotics will be the instrument of the future. In 2016, three scientists were awarded the Nobel Prize in Chemistry for their development of "the world's smallest machines," nano "elevators" and minuscule motors. Since then, the scientific experiments have progressed to the point where applicable devices are moving closer to actually being implemented.
Bionaut's technology was initially developed by a research team lead by Peer Fischer, head of the independent Micro Nano and Molecular Systems Lab at the Max Planck Institute for Intelligent Systems in Stuttgart, Germany. Fischer is considered a pioneer in the research of nano systems, which he began at Harvard University more than a decade ago. He and his team are advising Bionaut Labs and have licensed their technology to the company.
"The hope is that we can develop a vehicle to transport medication deep into the body," says Max Planck scientist Tian Qiu, who leads the cooperation with Bionaut Labs. He agrees with Shpigelmacher that the Bionaut's size is perfect for transporting medication loads and is researching potential applications for even smaller nanorobots, especially in the eye, where the tissue is extremely sensitive. "Nanorobots can sneak through very fine tissue without causing damage."
In "Fantastic Voyage," Raquel Welch's adventures inside the body of a dissident scientist let her swim through his veins into his brain, but her shrunken miniature submarine is attacked by antibodies; she has to flee through the nerves into the scientist's eye where she escapes into freedom on a tear drop. In reality, the exit in the lab is much more mundane. The Bionaut simply leaves the body through the same port where it entered. But apart from the dramatization, the "Fantastic Voyage" was almost prophetic, or, as Shpigelmacher says, "Science fiction becomes science reality."
This article was first published by Leaps.org on April 12, 2021.
How the Human Brain Project Built a Mind of its Own
In 2013, the Human Brain Project set out to build a realistic computer model of the brain over ten years. Now, experts are reflecting on HBP's achievements with an eye toward the future.
In 2009, neuroscientist Henry Markram gave an ambitious TED talk. “Our mission is to build a detailed, realistic computer model of the human brain,” he said, naming three reasons for this unmatched feat of engineering. One was because understanding the human brain was essential to get along in society. Another was because experimenting on animal brains could only get scientists so far in understanding the human ones. Third, medicines for mental disorders weren’t good enough. “There are two billion people on the planet that are affected by mental disorders, and the drugs that are used today are largely empirical,” Markram said. “I think that we can come up with very concrete solutions on how to treat disorders.”
Markram's arguments were very persuasive. In 2013, the European Commission launched the Human Brain Project, or HBP, as part of its Future and Emerging Technologies program. Viewed as Europe’s chance to try to win the “brain race” between the U.S., China, Japan, and other countries, the project received about a billion euros in funding with the goal to simulate the entire human brain on a supercomputer, or in silico, by 2023.
Now, after 10 years of dedicated neuroscience research, the HBP is coming to an end. As its many critics warned, it did not manage to build an entire human brain in silico. Instead, it achieved a multifaceted array of different goals, some of them unexpected.
Scholars have found that the project did help advance neuroscience more than some detractors initially expected, specifically in the area of brain simulations and virtual models. Using an interdisciplinary approach of combining technology, such as AI and digital simulations, with neuroscience, the HBP worked to gain a deeper understanding of the human brain’s complicated structure and functions, which in some cases led to novel treatments for brain disorders. Lastly, through online platforms, the HBP spearheaded a previously unmatched level of global neuroscience collaborations.
Simulating a human brain stirs up controversy
Right from the start, the project was plagued with controversy and condemnation. One of its prominent critics was Yves Fregnac, a professor in cognitive science at the Polytechnic Institute of Paris and research director at the French National Centre for Scientific Research. Fregnac argued in numerous articles that the HBP was overfunded based on proposals with unrealistic goals. “This new way of over-selling scientific targets, deeply aligned with what modern society expects from mega-sciences in the broad sense (big investment, big return), has been observed on several occasions in different scientific sub-fields,” he wrote in one of his articles, “before invading the field of brain sciences and neuromarketing.”
"A human brain model can simulate an experiment a million times for many different conditions, but the actual human experiment can be performed only once or a few times," said Viktor Jirsa, a professor at Aix-Marseille University.
Responding to such critiques, the HBP worked to restructure the effort in its early days with new leadership, organization, and goals that were more flexible and attainable. “The HBP got a more versatile, pluralistic approach,” said Viktor Jirsa, a professor at Aix-Marseille University and one of the HBP lead scientists. He believes that these changes fixed at least some of HBP’s issues. “The project has been on a very productive and scientifically fruitful course since then.”
After restructuring, the HBP became a European hub on brain research, with hundreds of scientists joining its growing network. The HBP created projects focused on various brain topics, from consciousness to neurodegenerative diseases. HBP scientists worked on complex subjects, such as mapping out the brain, combining neuroscience and robotics, and experimenting with neuromorphic computing, a computational technique inspired by the human brain structure and function—to name just a few.
Simulations advance knowledge and treatment options
In 2013, it seemed that bringing neuroscience into a digital age would be farfetched, but research within the HBP has made this achievable. The virtual maps and simulations various HBP teams create through brain imaging data make it easier for neuroscientists to understand brain developments and functions. The teams publish these models on the HBP’s EBRAINS online platform—one of the first to offer access to such data to neuroscientists worldwide via an open-source online site. “This digital infrastructure is backed by high-performance computers, with large datasets and various computational tools,” said Lucy Xiaolu Wang, an assistant professor in the Resource Economics Department at the University of Massachusetts Amherst, who studies the economics of the HBP. That means it can be used in place of many different types of human experimentation.
Jirsa’s team is one of many within the project that works on virtual brain models and brain simulations. Compiling patient data, Jirsa and his team can create digital simulations of different brain activities—and repeat these experiments many times, which isn’t often possible in surgeries on real brains. “A human brain model can simulate an experiment a million times for many different conditions,” Jirsa explained, “but the actual human experiment can be performed only once or a few times.” Using simulations also saves scientists and doctors time and money when looking at ways to diagnose and treat patients with brain disorders.
Compiling patient data, scientists can create digital simulations of different brain activities—and repeat these experiments many times.
The Human Brain Project
Simulations can help scientists get a full picture that otherwise is unattainable. “Another benefit is data completion,” added Jirsa, “in which incomplete data can be complemented by the model. In clinical settings, we can often measure only certain brain areas, but when linked to the brain model, we can enlarge the range of accessible brain regions and make better diagnostic predictions.”
With time, Jirsa’s team was able to move into patient-specific simulations. “We advanced from generic brain models to the ability to use a specific patient’s brain data, from measurements like MRI and others, to create individualized predictive models and simulations,” Jirsa explained. He and his team are working on this personalization technique to treat patients with epilepsy. According to the World Health Organization, about 50 million people worldwide suffer from epilepsy, a disorder that causes recurring seizures. While some epilepsy causes are known others remain an enigma, and many are hard to treat. For some patients whose epilepsy doesn’t respond to medications, removing part of the brain where seizures occur may be the only option. Understanding where in the patients’ brains seizures arise can give scientists a better idea of how to treat them and whether to use surgery versus medications.
“We apply such personalized models…to precisely identify where in a patient’s brain seizures emerge,” Jirsa explained. “This guides individual surgery decisions for patients for which surgery is the only treatment option.” He credits the HBP for the opportunity to develop this novel approach. “The personalization of our epilepsy models was only made possible by the Human Brain Project, in which all the necessary tools have been developed. Without the HBP, the technology would not be in clinical trials today.”
Personalized simulations can significantly advance treatments, predict the outcome of specific medical procedures and optimize them before actually treating patients. Jirsa is watching this happen firsthand in his ongoing research. “Our technology for creating personalized brain models is now used in a large clinical trial for epilepsy, funded by the French state, where we collaborate with clinicians in hospitals,” he explained. “We have also founded a spinoff company called VB Tech (Virtual Brain Technologies) to commercialize our personalized brain model technology and make it available to all patients.”
The Human Brain Project created a level of interconnectedness within the neuroscience research community that never existed before—a network not unlike the brain’s own.
Other experts believe it’s too soon to tell whether brain simulations could change epilepsy treatments. “The life cycle of developing treatments applicable to patients often runs over a decade,” Wang stated. “It is still too early to draw a clear link between HBP’s various project areas with patient care.” However, she admits that some studies built on the HBP-collected knowledge are already showing promise. “Researchers have used neuroscientific atlases and computational tools to develop activity-specific stimulation programs that enabled paraplegic patients to move again in a small-size clinical trial,” Wang said. Another intriguing study looked at simulations of Alzheimer’s in the brain to understand how it evolves over time.
Some challenges remain hard to overcome even with computer simulations. “The major challenge has always been the parameter explosion, which means that many different model parameters can lead to the same result,” Jirsa explained. An example of this parameter explosion could be two different types of neurodegenerative conditions, such as Parkinson’s and Huntington’s diseases. Both afflict the same area of the brain, the basal ganglia, which can affect movement, but are caused by two different underlying mechanisms. “We face the same situation in the living brain, in which a large range of diverse mechanisms can produce the same behavior,” Jirsa said. The simulations still have to overcome the same challenge.
Understanding where in the patients’ brains seizures arise can give scientists a better idea of how to treat them and whether to use surgery versus medications.
The Human Brain Project
A network not unlike the brain’s own
Though the HBP will be closing this year, its legacy continues in various studies, spin-off companies, and its online platform, EBRAINS. “The HBP is one of the earliest brain initiatives in the world, and the 10-year long-term goal has united many researchers to collaborate on brain sciences with advanced computational tools,” Wang said. “Beyond the many research articles and projects collaborated on during the HBP, the online neuroscience research infrastructure EBRAINS will be left as a legacy even after the project ends.”
Those who worked within the HBP see the end of this project as the next step in neuroscience research. “Neuroscience has come closer to very meaningful applications through the systematic link with new digital technologies and collaborative work,” Jirsa stated. “In that way, the project really had a pioneering role.” It also created a level of interconnectedness within the neuroscience research community that never existed before—a network not unlike the brain’s own. “Interconnectedness is an important advance and prerequisite for progress,” Jirsa said. “The neuroscience community has in the past been rather fragmented and this has dramatically changed in recent years thanks to the Human Brain Project.”
According to its website, by 2023 HBP’s network counted over 500 scientists from over 123 institutions and 16 different countries, creating one of the largest multi-national research groups in the world. Even though the project hasn’t produced the in-silico brain as Markram envisioned it, the HBP created a communal mind with immense potential. “It has challenged us to think beyond the boundaries of our own laboratories,” Jirsa said, “and enabled us to go much further together than we could have ever conceived going by ourselves.”